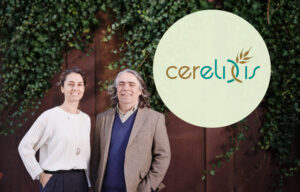
Neural pathways
Exploring the diverse routes by which EMBL scientists are driving forward neurobiology
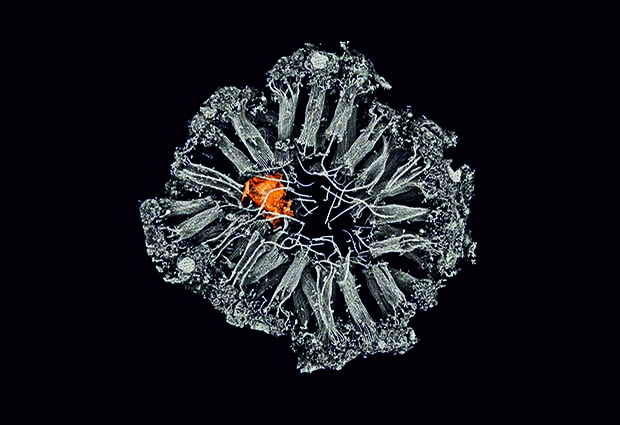
Making sense of the human experience has long been a quest of philosophers and scientists. How do we perceive the world around us? Why do we behave in a certain way in response to a stimulus? How do our neurons organise themselves into circuits and networks to keep our internal supercomputers running at maximum efficiency – and what happens when a bug gets into the operating system? These questions are typical of the conundrums exercising the minds of neurobiologists at EMBL.
Computer brain
“The brain is just too complex!” says Hiroki Asari, group leader at EMBL Rome. He smiles now at the recollection of his “undergrad naivety” as a physics and maths student: “I had this idea that I could simulate the brain using a computer. That was a big motivation for getting into neurobiology.”
Asari was nearing the end of his PhD in systems neuroscience at Cold Spring Harbor Laboratory in the US when a realisation triggered a shift in his focus and methodology: his computer work alone – modelling how the brain processes sound – couldn’t tell him what was actually going on inside the brain. He decided to switch from the auditory to the visual system, to explore how the retina converts light signals into electrical neuronal signals – the first stage of sensory processing. “Unless we understand this, we can’t understand the brain,” he says.
Asari now complements his computational work with experimental approaches in the lab, to study how the retina works in vivo. “We’re trying to answer a couple of big questions,” he says. “What kinds of signals are the eyes sending to the brain, and how are those signals affected by different internal or behavioural states? If you’re hungry, for example, a cookie looks a lot more attractive than if you’re not.”
Mouse brain
Working with mouse models, Asari uses fluorescent proteins to study visual responses to a light stimulus. These proteins – delivered to the neurons via an injected virus – make it possible to tell whether a neuron is active or not. If it’s active, it shines.

But we humans are not mouse-brained. Can Asari’s work tell us anything relevant about our own neural function? “I believe so,” he says. “The organisation of the retinal circuitry is fairly preserved across species, among mammals especially. So we think our basic findings in mice can be applied to humans by understanding the principles underlying neural circuit functions. This is where a computational approach is very useful – we can try to build a model to generalise the function of the retina across different species.”
Threat and response
Cornelius Gross, Asari’s colleague and fellow group leader at EMBL Rome, is also looking to mice to help unravel some of the mysteries of the human condition. His interest lies in behaviour, and in understanding the subtle differences between individuals in how mice – and people – respond to certain stimuli.
“I’ve always been interested in behaviours associated with defence, fear and anxiety – I want to understand how our neural circuits encode and control these behaviours,” says Gross. He’s focusing on the hypothalamus – an old part of the brain, in evolutionary terms – which is associated with some fundamental behaviours, including fear.
As it turns out, there’s not just a single fear circuit in the brain that determines how we respond to threatening stimuli. The type of threat matters. Gross has studied two fundamental types of fear: fear of predators, and fear as a response to social threats, such as bullying or aggression. “We found that there are two separate, independent pathways for handling these two types of threat. Even if the final outputs – the fear responses – are the same, they’re encoded by two dedicated circuits in the hypothalamus,” he says. This hadn’t been shown before.
Another finding to emerge from Gross’s work is that there’s also an encoding of space, or territory, when it comes to social interactions. Mice are naturally territorial, and it seems that their awareness of being in a safe place versus being in a threatening place is linked to their neuronal activity. “In other words,” Gross explains, “a place in the brain that was considered to be only social and instinctive suddenly becomes much more sophisticated – it has a map of your world, of your territory.”
Eat me
A smaller but no less fascinating part of the work in Gross’s lab focuses on microglia. These are cells that are born not in the brain but in the yolk sac – a membrane connected to the embryo in early development. They then migrate into the brain, where – as shown by in vivo imaging – they’re continuously moving around. But what are they doing?

“We don’t really know,” says Gross. “This is a field that’s in its infancy.” What he does know is that during the early period of brain development, when most of the neural connectivity is formed, neurons actively send out signals to microglia. “These microglia have been described as phagocytic: they eat things,” Gross continues. This led him to a hypothesis: the microglia eat synapses – the connections between neurons – that need to be removed for some reason.
Gross and his team subsequently discovered that microglia don’t eat synapses. Not exactly. Rather, they seem to ‘nibble’ parts of synapses and neurons. It’s not yet clear what this nibbling does, but it may promote new contacts between neurons. Also unclear is what determines which neurons get nibbled. “What’s the ‘eat me’ signal?” Gross wonders. “That’s what we want to find out next.”
Beneath the surface
EMBL Rome is a hub for EMBL’s neurobiology research, but scientists at other EMBL sites are also active in the field. Kyung-Min Noh – a group leader at EMBL Heidelberg – broke the mould when she decided to become a scientist, in a family more inclined towards the arts. It’s perhaps unsurprising, then, that it was aesthetics that first lured her into the field of neurobiology. “When you look at neurons under the microscope, you can’t but fall in love,” she says. “They’re beautiful.”
However, Noh – a group leader at EMBL Heidelberg – soon began to look beyond the morphology of the neuron, to what was going on in the nucleus while neurological pathways were being developed in the brain. She found there was a link between neurodevelopmental disorders and chromatin – the mass of DNA and proteins in the cell nucleus, which plays a role in gene expression. “In the lab, we’re trying to understand how this works mechanistically – the molecular mechanisms that link genetic mutations and changes in gene expression to changes in the chromatin,” she says.
On individuality
One aspect of the research carried out in Noh’s lab focuses on mutations in people with autism spectrum disorders: “We know the genetic mutations happen in the chromatin. They’re all under the umbrella of autism spectrum disorders, but individually they’re very different. We’re trying to understand the differences between these individual molecules, which present behaviourally as autism spectrum disorders. What exactly is happening inside the cells?”

To find out, Noh uses the CRISPR gene editing tool to introduce mutations into mouse cells or human induced pluripotent stem (iPS) cells – cells that are generated from adult cells and can differentiate into many different cell types. She collaborates with fellow Heidelberg group leaders Judith Zaugg and Christoph Müller, working with Zaugg’s group on genomic sequencing and bioinformatic analysis, and with Müller’s group to understand the molecular structure of the chromatin-associated protein complex. “This collaborative effort means that we can apply the state of the art to all aspects of the work,” says Noh.
How proteins signal
Andrew McCarthy, a team leader at EMBL Grenoble, is also interested in molecular structures in the context of neurobiology. Specifically, he wants to uncover the underlying mechanism of the Slit and Roundabout (Slit–Robo) cell signalling pathway – essentially, how signals from outside a cell are transmitted to the inside. “These are two proteins. Slit is secreted by specialised glial cells in the nervous system, and Robo is the cognate receptor in the neuronal cell membrane. They interact with each other – they ‘talk’ to each other – and we’re trying to figure out how they signal once they interact,” McCarthy says.
These proteins are expressed in the early stages of embryonic development, when the neural networks are being laid down – connecting the eyes and the muscles to the brain, for example, for the visual and motor systems. “A lot of these developmental processes are very dynamic,” says McCarthy, “so the proteins are sensing their environment rapidly, and cells infer how to grow or retract in different areas.”

Many cancers and other diseases have been linked to these developmental proteins. “Not just Slit and Roundabout,” McCarthy adds. “There’s a whole plethora of them.” The link is particularly strong in the case of metastatic cancer cells because such proteins can inform cells to migrate and react to their environment. If unregulated, the proteins can induce primary tumour cells to break away and move to other parts of the body.
These are difficult proteins to work with in the lab. “They’re large, very flexible, and hard to handle,” says McCarthy. But technological developments are making things easier. Improvements in the beamlines at the European Synchrotron Radiation Facility (ESRF), with which EMBL Grenoble has close ties, mean that data can be collected faster, and from smaller crystals. McCarthy would also like to take advantage of developments in electron and light microscopy. “We know what these proteins look like – roughly. Now we need to see them in context, moving to or from the cell surface.”
Origin and evolution
With these scientists following their curiosity down diverse lines of inquiry, our knowledge about the most complex system in the human body is ever increasing. Yet there remains a great mystery to be solved: how did neurons and nervous systems evolve? This is the question that intrigues EMBL Heidelberg group leader Detlev Arendt.
“Humans have always wanted to know where we come from, and this is what we’d like to find out,” Arendt says. “We want to trace evolutionary paths towards neurons and nervous systems. So we look at animals that are more remote on the animal tree – animals with simple versions of a nervous system, or those that don’t have a nervous system because they branched off earlier.”
Looking to the ocean for answers
Landlocked Heidelberg, hundreds of kilometres from the ocean, is an unlikely location for a marine biology lab. Yet Arendt’s lab is full of tanks in which a variety of marine species reside in water from the North Sea. These include sponges, molluscs, and the marine worm Platynereis dumerilii – a relative of the earthworm, with a typical rope-ladder-like nervous system. But what can we learn from these species?
Arendt explains: “We’re interested in ancestors, and this famous urbilaterian – the last common ancestor of all animals with bilateral symmetry. So we’re working on a lot of informative species to try to reconstruct that animal by comparison. If a trait – be it a gene, a cell type, or a body part – exists in many species using shared information, it’s likely that this feature existed in the last common ancestor.”

Arendt and collaborators Yannick Schwab, Anna Kreshuk and Christian Tischer at EMBL Heidelberg – who share his enthusiasm for neurobiology – recently made a fascinating discovery in sponges – our most remote cousins in the animal kingdom. Although they don’t have a nervous system, sponges have a lot of the same proteins found at the synapses – the connections between neurons – in other animals. The scientists wanted to find out what these synaptic proteins were doing, and in the course of their investigations they discovered two cell types highly enriched in synaptic proteins: a migratory cell type with a lot of extensions – similar to a neuron’s axons – that contacts cells in the choanocyte chamber, which acts as a water pump, bringing oxygen and nutrients into the sponge. Their next step is to investigate where precisely the proteins are located in the cells, and how they function in cellular communication.
EMBL-flavoured neurobiology
Neurobiology research at EMBL continues to take shape in interesting directions, and Cornelius Gross is emphatic that EMBL has something unique to offer in this sphere. “EMBL is great at genomics and gene expression, it’s great at imaging, and it’s great at structural biology. Neurobiology at EMBL should take advantage of those features. That’s the vision – a kind of EMBL-flavoured neurobiology.”