Read the latest Issue
20 years in the making
“This has taken 20 years of my life,” smiles Head of EMBL Grenoble Stephen Cusack, “and for the first 15 years we had almost no results!” Cusack’s perseverance, determination and vision have now been duly rewarded as he and his team publish the first crystal structure of an influenza polymerase this week in the journal Nature.
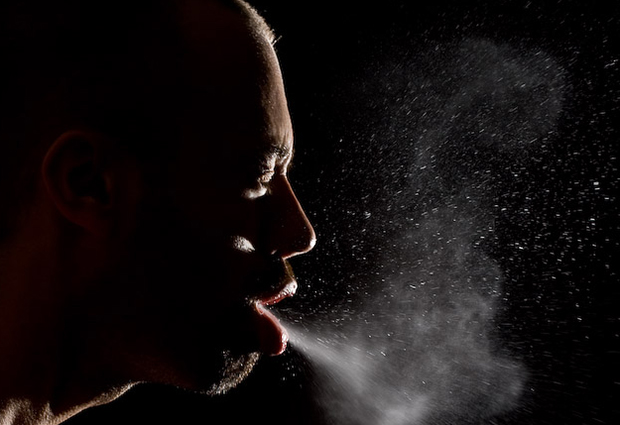
Influenza is a major infectious disease, but despite its common occurrence the virus is still not really well understood at the molecular level. The results published this week represent a revolution in the field of flu research and will help to answer a vast number of questions about the virus’ replication but, as Cusack says, “we also have a lot of new questions too.”
As the days grow shorter and colder, the annual wave of coughs, colds and bugs lurks around the corner waiting to work its way through schools and workplaces and send us to our beds. And while the majority of us will be able to shake off a bout of the flu without any medication other than hot drinks, painkillers and rest, for some it represents a real health risk. For babies, the elderly and patients with weakened immune systems, it can mean serious health complications such as pneumonia, or even prove fatal. Even during the relatively mild winter of 2013/2014, the Robert Koch Institute reported an estimated 430,000 cases of flu-associated sick leave and some 3100 cases of hospitalisations in Germany alone.
Influenza A strains result in some “3-5 million cases of severe illnesses and about 250 to 500 thousand deaths” worldwide
Not all flu is created equal
The flu – or influenza to give it its full name – is a virus that comes in two main guises. Influenza A infects humans, certain other mammals (including pigs, horses, bats and seals) and birds (such as water birds and poultry) and gives rise to seasonal epidemics and occasional serious pandemics. Influenza B infects only humans and, while causing seasonal sickness, does not present the same global health risk as influenza A. Although infection generally occurs via the transmission of droplets carried on the air from one person to the next, some occasional – but severe – highly pathogenic bird strains have been known to transmit to humans. In 1918, a worldwide pandemic caused by a strain that made this leap from birds to humans, probably via pigs, killed some 50 million people worldwide. In 2009, a variant of the same strain, H1N1, caused the so-called ‘swine flu’ pandemic that originated in pigs, resulting in some 284,500 deaths worldwide. Highly infectious avian strains of influenza A, H5N1 and H7N9, have recently caused several serious outbreaks, devastating poultry industries in Asia and infecting a significant number of people. The World Health Organization estimates that annual epidemics of influenza A strains result in some “3-5 million cases of severe illnesses and about 250 to 500 thousand deaths” worldwide.
Although the virus has been well studied, annual outbreaks have been documented and surveyed, and the high economic cost has been chronicled, there is still no cure and eradication of the virus is as yet inconceivable. Seasonal vaccinations are recommended for high-risk groups such as the elderly and health workers, but vaccines comprise only those strains predicted to be the most prevalent in that season, and so do not protect against other variants. Current antiviral drugs, such as Tamiflu, can reduce and alleviate the symptoms and prevent serious health consequences, but they cannot completely cure the patient and the virus will often develop resistance to these treatments.

Final piece of the puzzle
Twenty years ago, Stephen Cusack started working on the polymerase of influenza A – one of the ‘holy grails’ of influenza research. “That’s the one everyone really wants to understand,” Cusack says, ”since it’s the essential machine that replicates the viral genome.” When the virus infects and enters the host cell, it sets about reproducing: replicating its genomic material and transcribing it so that it can be read by the host’s protein production machinery, which is then tricked into making viral proteins. It is the polymerase that is responsible for both the transcription and replication of the virus and is therefore crucial to its virulence and survival. Understanding how this key enzyme works would pave the way for the development of new antiviral drugs.
It’s the essential machine that replicates the viral genome
The plan was to resolve the structure of the enzyme using structural biology approaches, such as X-ray crystallography. “The polymerase has been known about for some 40 years,” explains Cusack, “so there is 40 years’ worth of literature on biochemical and genetic studies trying to understand how it all works.” Having the structure of the enzyme would help to put all previous studies into context and finish the puzzle. And the polymerase seems to be particularly puzzling. It consists of three entwined subunits, making it difficult to decipher and to find out which subunit does what. “Not only does it bind viral RNA, transcribing it to messenger RNA, but it also replicates the RNA to be packaged into new virus particles,” Cusack emphasises. How can it do both things with only one active site? How does it switch from one task to the other? How does it bind to viral RNA in the first place? These questions have long been debated in the field, but without a complete structure, it has been difficult to put everything into place.
Switching to plan B
To determine the enzyme’s structure, the scientists needed samples in crystalline form: repetitive arrangements of the molecule that they could then analyse by measuring how they interfered with X-rays shone onto the sample. To produce crystals, the first task is to obtain sufficient amounts – measured in milligrams – of pure polymerase. But for the polymerase of human or avian influenza A virus, this turned out be impossible. Instead, from 2007 Cusack’s group and collaborators contributed several significant insights into the workings of the polymerase by solving the structures of isolated, but functionally important, fragments of the enzyme.
These fragments included the two domains of the polymerase that are required for the unique ‘cap-snatching’ transcription mechanism. Both these domains, if blocked by small molecules, inhibit the working of the polymerase and are therefore good targets for anti-flu drug development, which Cusack has pursued with a start-up company (Savira) and Roche Pharmaceuticals.

But the structure of the entire polymerase remained elusive. Cusack and his team decided to change tack and started looking at influenza B instead. “This was one of a number of significant breakthroughs,” he says. Although influenza B does affect humans, it only makes up a small proportion of seasonal epidemic infections and its slow rate of change means that most people acquire some state of immunity in their childhood. By contrast, the constant and rapid evolution seen in the Influenza A virus makes it harder for the immune system and health agencies to keep up.
Having made the switch to influenza B, and with the help of techniques developed by group leader Imre Berger, also at EMBL Grenoble, Cusack and his team were soon able to produce enough of the enzyme for crystallisation experiments. Once they had managed to make crystals, the structure soon followed: “It all went very quickly,” says Cusack, still surprised by the dramatic turn of events. Shortly after, the group also managed to express a strain of influenza A from bats that was only discovered recently and is genetically very similar to human A strains. Key to the success of this project were developments in technology: “The high-intensity X-ray beamlines at the ESRF, equipped with state-of-the-art detectors available by this time, were crucial for getting high-quality crystallographic data from weakly diffracting crystals of the large polymerase complex,” says Cusack. “We couldn’t have got the data at this resolution without them.”
The big picture
Now, with the information from the atomic structures of the influenza A and B polymerases, Cusack and his team can finally propose a more complete and detailed description of how it works. The new data show that the three subunits that make up the enzyme are heavily intertwined, with all three units intricately involved in the function of the polymerase. “Now that we can see how everything fits together, it’s no wonder that the biochemical results produced over the past 40 years have been confusing,” says Cusack.
The different structures also capture the polymerase in different states of activity, which helps to show the dynamics of the system. The team had already determined the structure of the subunit that ‘snatches’ a specific piece of host-cell RNA needed to kick-start the production of viral proteins. Now that they know the structure of the whole polymerase, they can see how that part of the enzyme moves relative to the others, revealing that it is able to rotate by a large angle to bring the ‘snatched’ piece of RNA in line with the polymerase’s active site so that transcription can begin.
Although the structures are from different influenza species, Cusack does not believe that this is significant when it comes to understanding how the polymerase works, and how we might be able to stop it in its tracks. “The functional areas of the polymerase are very similar, and we think this information will also shed light on understanding how the polymerases of other related viruses work – such as that from the Ebola virus, for example,” says Cusack.
There are a whole lot more questions to be answered, but at least we are in a much better starting position
So, what next? “We need to crystallise the machine in different functional states so we can be sure how it works,” says Cusack. “We have already begun high-throughput screening to try to find inhibitors for the active site based on this new information,” he adds. Although the structures reveal a lot about how the viral polymerase works, the living cells that the virus infects are a lot more complex than the purified polymerase used in the laboratory. “There are a lot of other players in the infected host cell,” says Cusack. “What role do the host proteins play in helping, or hindering, the polymerase working? The viral RNA genome is protected by a coat of viral nucleoproteins. How are these removed and put back on again to allow the passage of the polymerase? There are a whole lot more questions to be answered, but at least we are in a much better starting position to answer them.”