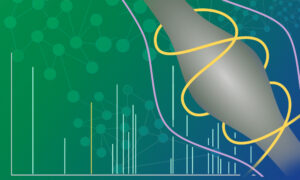
Read the latest Issue
EMBL Group Leaders Maria Bernabeu and Eva Kowalinski, and EMBL alumnus Freddy Frischknecht are working on tropical diseases which cause 700,000 deaths every year.
By Mylène André and Luca Tancredi Barone
The World Health Organization (WHO) reports that more than one-sixth of all infectious diseases are vector-borne diseases – in other words, diseases in which a pathogen is transmitted from one host to another by an intermediate organism, known as a vector. Many of these vectors are insects that feed on blood, such as mosquitoes or ticks. Vector-borne diseases result in hundreds of millions of cases and around 700,000 deaths each year. These diseases tend to be concentrated in tropical and subtropical areas, and they have a disproportionate impact on the poorest communities, which may suffer from factors such as a lack of shelter, poor sanitation, or limited access to healthcare.
Given the serious impact of these diseases on human health, and the severe economic burdens they cause, there is a pressing need for research to better understand the processes of infection and the biology of the pathogens involved, to enable the development of new and more effective methods of prevention or treatment.
The deadliest vector-borne disease is malaria, which claims 400,000 lives each year, most of them in Africa. Malaria depends on two organisms, both of which are necessary for the disease to attack: a female Anopheles mosquito and a tiny but very sophisticated single-celled parasite called Plasmodium. The cycle begins when a mosquito bites a human to feed on their blood. If the insect hosts the parasite, it carries an infective form called a sporozoite, which can be passed on to its human victim. Once the sporozoite reaches the bloodstream, it moves towards the liver. Here it can reproduce and create thousands of offspring of another form, called merozoites. These attack red blood cells, where they reproduce. The red blood cells eventually burst, liberating yet more merozoites, and this is when patients experience fever and the other symptoms of malaria.
EMBL alumnus Freddy Frischknecht, a professor at Heidelberg University, carries out research on Plasmodium. He focuses especially on the extraordinary method the parasite uses to move around inside its host. “Everybody assumed that the sporozoite was injected by the mosquito directly into the blood, but instead they’re injected into the skin,” he explains. “From there, Plasmodium looks for a blood vessel.”
Research in Freddy’s group revealed how the parasites move ten times faster than any other migrating cell in the body. “The fastest moving cell in our body is the neutrophil cell, part of the immune system. It’s as if the fastest police car runs at a tenth of the speed of the villain,” he says.
He and his group have made important steps in understanding how Plasmodium manages to be that fast. They found that the parasite tries to avoid sticking to a substrate. Instead it glides, which it can do thanks to an interplay between filaments of a protein called actin, which determine the shape and movement of the cell’s surface. Recent work led by group leader Christian Löw at EMBL Hamburg has provided further insights into the structure of the proteins that enable this gliding motion (p. 11).
Frischknecht is working on a type of Plasmodium that attacks mice: his group discovered that if you modify the gene sequence coding for some proteins involved in the migration mechanism, the parasite could still infect mice, but the mice would survive. “We think this is because the parasite then multiplies more slowly in the blood, and the immune system is better at controlling fewer parasites,” he says. His goal now is to generate a human-infecting parasite that grows more slowly than the normal one, but can still be transmitted by a mosquito. Just as in mice, the hope is that the human immune system would be capable of fighting off this form of the parasite, enabling the host to gain immunity to other strains of Plasmodium that infect humans. This is the kind of novel approach that’s needed to tackle malaria, since designing a conventional vaccine – using proteins on the parasite’s surface – is extremely difficult, as there are many such proteins and they undergo significant changes during the parasite’s life cycle. There is currently only one approved malaria vaccine, which offers protection in only about 30% of cases and for a limited time.
At EMBL Barcelona, group leader Maria Bernabeu is tackling malaria from a different perspective. Her research – for which she was recently awarded a €1.5m ERC Starting Grant – focuses on cerebral malaria, a deadly complication of the disease that involves severe neurological symptoms, and is responsible for approximately three-quarters of malaria deaths worldwide.
“One of the things that prevents us from understanding how this disease evolves is that we don’t have direct access to the brain until after a patient has died, so we do not understand its progression,” Maria explains. A collaboration between her group and clinicians at Ispat General Hospital (IGH) in Rourkela, India, will help overcome this problem. IGH is one of the few places in the world using MRI scanning to study the brains of patients with malaria, offering a way to closely monitor the development of the disease in living patients.
The brain is usually protected from infection by the blood–brain barrier (BBB). Maria’s group is studying how cerebral malaria breaks down this barrier. They are growing cells on collagen scaffolds to recreate living human blood microvessels of the same size and shape as the real ones in the BBB. “Through this blood vessel tree, we can flow blood cells – both healthy ones, and those infected with malaria,” Maria says. “This allows us to recreate aspects of the human pathology in a dish – using human cells and the human-specific version of the parasite – to better understand the mechanism of cerebral malaria.”
This is the first time anyone has used artificial blood vessels to study malaria, and the methods being developed in Maria’s group could bring us an important step closer to tackling this disease. “If you find a strategy to prevent the complications of cerebral malaria,” says Maria, “you can save many lives.”
At EMBL Grenoble, group leader Eva Kowalinski and members of her lab are carrying out research on Trypanosoma: a genus of single-celled insect-transmissible parasites that can cause human and animal diseases. The Kowalinski group studies RNA – a molecule that plays an important role in carrying genetic information to be converted into a gene product such as a protein. The group uses structural biology, biochemistry, and cell biology methods to study the mechanisms of protein–RNA interactions and RNA metabolism: the ways in which RNA molecules are produced, modified, processed, and broken down.
Members of the group study RNA molecules in Trypanosoma parasites to understand how the genetic information is processed, modified, or even re-written. These pathways differ significantly between Trypanosoma and humans. “We study in particular how the protein complexes involved differ from those in human cells. Trypanosomes have very special forms of RNA processing. From a fundamental research aspect, it’s really interesting to understand how it works,” says Eva, whose group recently received a grant from the French National Research Agency (ANR) for this research.
Parasites of the species Trypanosoma brucei are transmitted between humans or animals by the bite of an insect vector, the tsetse fly, when taking a blood meal. Infection with the parasite causes so-called sleeping sickness in humans and a disease called nagana in animals. Sleeping sickness produces a range of symptoms such as fever, swelling of the lymph nodes, and subsequently neurological symptoms including sleep disturbances. It is fatal if left untreated, and is a severe health threat in sub-Saharan Africa, a region which is also widely affected by other diseases and economic hardship. According to WHO, the number of infected people has dropped significantly over the past 50 years, thanks to a sustained effort to tackle this disease. However, its diagnosis and treatment are still very complex, and there is no vaccine.
There is a growing quantity of cell biology data demonstrating the importance of the RNA processing pathways for proliferation of trypanosomes. Targeting these pathways with drugs could prevent the parasite from reproducing or – in the best case – could kill the parasite. By determining the structures of the proteins involved in trypanosomal RNA processing, scientists can begin to design drugs that specifically target only the proteins of the parasite and not the corresponding proteins – if they exist – in humans. The more a target differs from the human one, the more likely it is that a drug can work effectively without causing the kind of strong side-effects that are seen in current Trypanosoma treatments. “Understanding why the metabolism of this organism is different from ours will then be very useful for applied research to develop efficient drugs and find straightforward therapies,” says Eva.
The Kowalinski group uses a lab-safe strain of Trypanosoma brucei for their research. Fortunately, any results from studies on Trypanosoma brucei can be more widely applied to other Trypanosoma species and to another genus of parasites called Leishmania, which also cause an infectious tropical disease and process their RNAs in a similar way.
The impact of climate change on tropical and infectious diseases is hard to predict, but it seems certain that global warming will reshape the regions in which insects transmit infectious diseases. Due to the narrow temperature window that favours the proliferation of insect vectors, zones with a certain disease prevalence will probably move, and the insects might invade new regions that have been previously untouched. “If this happens, it will cause huge problems because the health systems and vector control infrastructure are not prepared for it,” says Eva. “The current COVID-19 crisis shows us the importance of fundamental research on infectious diseases. We already need to be thinking ahead to what could be the next global challenges. Rigorous research needs time. Investing in research on diseases that we predict could become more widespread in the coming years is really important, to avoid human catastrophes.”
Looking for past print editions of EMBLetc.? Browse our archive, going back 20 years.
EMBLetc. archive