Hundreds of tiny robots grow bio-inspired shapes
Scientists build self-organising features into robot swarms to study shape formation
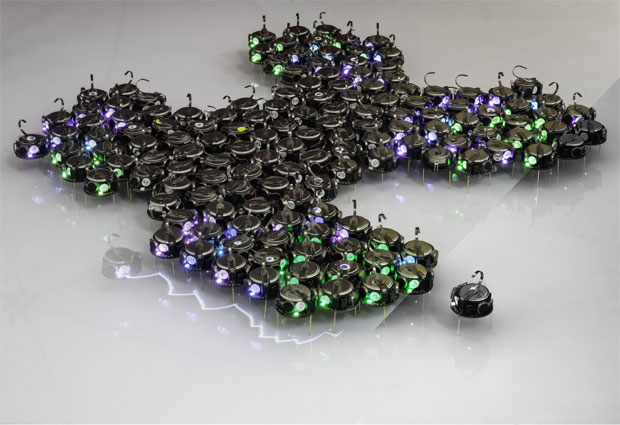
Hundreds of small robots can work in a team to create biology-inspired shapes – without an underlying master plan, purely based on local communication and movement. To achieve this, researchers from EMBL, CRG and Bristol Robotics Laboratory introduced the biological principles of self-organisation to swarm robotics. Science Robotics publishes the results on 19 December.
“We show that it is possible to apply nature’s concepts of self-organisation to human technology like robots,” says EMBL Barcelona group leader James Sharpe. “That’s fascinating because technology is very brittle compared to the robustness we see in biology. If one component of a car engine breaks down, it usually results in a non-functional car. By contrast when one element in a biological system fails, for example if a cell dies unexpectedly, it does not compromise the whole system, and will usually be replaced by another cell later. If we could achieve the same self-organisation and self-repair in technology, we can enable it to become much more useful than it is now.” Sharpe led the project – initiated at the Centre for Genomic Regulation (CRG) – together with Sabine Hauert at the University of Bristol.
Shape formation as seen in the robot swarms. Complete experiments lasted for three and a half hours on average. Inspired by biology, robots store morphogens: virtual molecules that carry the patterning information. The colours signal the individual robots’ morphogen concentration: green indicates very high morphogen values, blue and purple indicate lower values, and no colour indicates virtual absence of the morphogen in the robot. Each robot’s morphogen concentration is broadcasted to neighbouring robots within a 10 centimetre range. The overall pattern of spots that emerges drives the relocation of robots to grow protrusions that reach out from the swarm. See a longer video: https://youtu.be/bEm-fXkLw7g.
Turing’s rules
The only information that the team installed in the coin-sized robots were basic rules on how to interact with neighbours. In fact, they specifically programmed the robots in the swarm to act similarly to cells in a tissue. Those ’genetic’ rules mimic the system responsible for the Turing patterns we see in nature, like the arrangement of fingers on a hand or the spots on a leopard. In this way, the project brings together two of Alan Turing’s fascinations: computer science and pattern formation in biology.
The robots rely on infrared messaging to communicate with neighbours within a 10 centimetre range. This makes the robots similar to biological cells, as they too can only directly communicate with other cells physically close to them.
The swarm forms various shapes by relocating robots from areas with low morphogen concentration to areas with high morphogen concentration – called ‘turing spots’, which leads to the growth of protrusions reaching out from the swarm. “It’s beautiful to watch the swarm grow into shapes, it looks quite organic. What’s fascinating is there is no master plan, these shapes emerge as a result of simple interactions between the robots. This is different from previous work where the shapes were often predefined.” says Sabine Hauert.
Working with large robot swarms
It is impossible to study swarm behaviour with just a couple of robots. That is why the team used at least three hundred in most experiments. Working with hundreds of tiny robots is a challenge in itself. They were able to do this thanks to a special setup which makes it easy to start and stop experiments, and reprogram all the robots at once using light. Over 20 experiments with large swarms were done, with each experiment taking around three and a half hours.
Furthermore, just like in biology, things often go wrong. Robots get stuck, or trail away from the swarm in the wrong direction. “That’s the kind of stuff that doesn’t happen in simulations, but only when you do experiments in real life”, says Ivica Slavkov, who shares first authorship of the paper with Daniel Carrillo-Zapata.
All these details made the project challenging. The early part of the project was done in computer simulations, and it took the team about three years before the real robot swarm made its first shape. But the robots’ limitations also forced the team to devise clever, robust mechanisms to orchestrate the swarm patterning. By taking inspiration from shape formation in biology, the team was able to show that their robot shapes could adapt to damage, and self-repair. The large-scale shape formation of the swarm is far more reliable than each of the little robots, the whole is greater than the sum of the parts.
Potential for real world applications
While inspiration was taken from nature to grow the swarm shapes, the goal is ultimately to make large robot swarms for real-world applications. Imagine hundreds or thousands of tiny robots growing shapes to explore a disaster environment after an earthquake or fire, or sculpting themselves into a dynamic 3D structure such as a temporary bridge that could automatically adjust its size and shape to fit any building or terrain. “Because we took inspiration from biological shape formation, which is known to be self-organised and robust to failure, such swarm could still keep working even some robots were damaged.“ says Daniel Carrillo-Zapata. There is still a long way to go however, before we see such swarms outside the laboratory.
James Sharpe (EMBL Barcelona) led the Swarm-Organ project, which was initiated at the Centre for Genomic Regulation (CRG) when Sharpe was a group leader there. Sabine Hauert (Bristol Robotics Laboratory and University of Bristol) was the key senior collaborator. Other collaborators were Fredrik Jansson (currently employed at Centrum Wiskunde & Informatica – CWI) and Jaap Kaandorp (University of Amsterdam – UvA).
The research leading to these results has received funding from the European Union Seventh Framework Programme (FP7) under grant agreement n° 601062, and the EPSRC Centre for Doctoral Training in Future Autonomous and Robotic Systems (FARSCOPE) at the Bristol Robotics Laboratory.
Crear formas biológicas con cientos de robots diminutos
Científicos del EMBL, el CRG y la Universidad de Bristol crean características de autoorganización en enjambres de robots para estudiar la creación de formas
Cientos de pequeños robots pueden trabajar en equipo para crear formas de tipo biológico, sin un plan maestro subyacente, basándose puramente en la comunicación mutua y el movimiento. Para lograrlo, los investigadores introdujeron los principios biológicos de autoorganización en la robótica de enjambre. Science Robotics publica los resultados el 19 de diciembre.
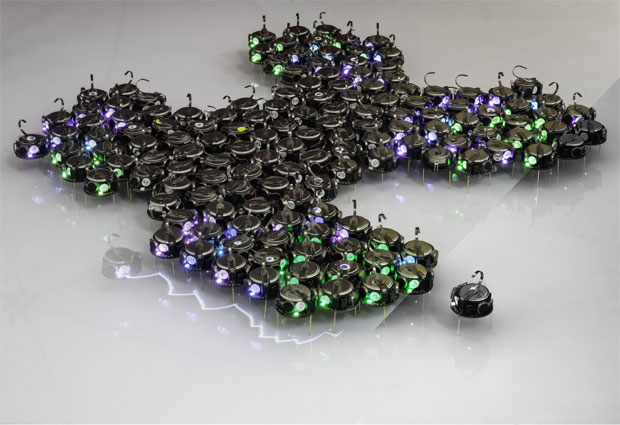
“Demostramos que es posible aplicar los conceptos de autoorganización de la naturaleza a la tecnología humana como los robots”, dice el líder del grupo del EMBL Barcelona, James Sharpe. “Esto es fascinante porque la tecnología es muy frágil en comparación con la robustez que vemos en la biología. Si un componente del motor de un coche se avería, normalmente da como resultado un coche que no funciona. En cambio, cuando un elemento de un sistema biológico falla, por ejemplo, si una célula muere repentinamente, no compromete todo el sistema. Y podría incluso ser reemplazada por otra célula posteriormente. Si pudiéramos lograr la misma tecnología de auto organización y auto reparación, podríamos conseguir que fuera mucho más potente de lo que es ahora”. Sharpe dirigió el proyecto, iniciado en el Centro de Regulación Genómica (CRG), junto con Sabine Hauert de la Universidad de Bristol.
Creación de formas como se ve en los enjambres de robots. Cada vídeo se acelera unas 100 veces. Los colores señalan la concentración morfógena del robot individual: verde indica valores morfógenos muy altos, azul y púrpura indican valores más bajos, y sin color indica ausencia virtual de morfógeno en el robot. Los morfógenos son las moléculas que llevan la información de la generación de patrones en los sistemas biológicos. Estos son imitados en los robots. La concentración de morfógenos en cada robot es transmitida a los robots vecinos en un radio de 10 centímetros. La forma del enjambre es impulsada por los valores del morfógeno y el patrón general. https://youtu.be/bEm-fXkLw7g
Video: reimpresa con permiso de Slavkov, I., Zapata D. C. et al., Science Robotics (2018).
Reglas de Turing
La única información que el equipo instaló en los robots – que tienen el tamaño de una moneda – fueron reglas básicas sobre cómo interactuar con los vecinos. De hecho, programaron los robots específicamente en un enjambre para actuar como las células en un tejido. Estas reglas “genéticas” imitan el sistema responsable de los patrones de Turing que vemos en la naturaleza, como la organización de los dedos en una mano o las manchas de un leopardo. De esta manera, el proyecto reúne dos de las fascinaciones de Alan Turing: la informática y la formación de patrones en la biología.
Los robots se comunican con los vecinos por infrarrojos en un radio de 10 centímetros. Esto hace que los robots sean similares a las células biológicas, ya que estas también se comunican directamente solo con otras células próximas físicamente.
El enjambre es capaz de crear varias formas trasladando robots de zonas con baja concentración de morfógenos a zonas con una alta concentración – llamadas “círculos Turing” – hecho que lleva a crear tentáculos en el enjambre de robots. “Es fantástico ver cómo el robot crea diferentes formas. Parece un proceso orgánico. Lo que es más fascinante es que no hay un plan preestablecido, las formas surgen como resultado de las interacciones entre los robots. Esto diferencia nuestro trabajo de investigaciones anteriores, donde a menudo las formas estaban predefinidas.” comenta Sabine Hauert.
Trabajar con grandes enjambres de robots
Es imposible estudiar el comportamiento de un enjambre con solo un par de robots. Por eso el equipo utilizó al menos trescientos en la mayoría de los experimentos. Trabajar con cientos de robots diminutos es un reto en sí mismo. El equipo de investigación lo llevo a cabo utilizando un método que facilita iniciar y detener el experimento, así como reprogramar todos los robots a la vez utilizando luz. En total se realizaron unos 20 experimentos con un gran número de robots, con una duración aproximada de 3 horas por experimento.
Es más, al igual que en la biología, a veces las cosas salen mal. Como las células, los robots se quedan atascados, o se separan del enjambre en la dirección equivocada. “Este es el tipo de cosas que no pasan en las simulaciones, sino cuando realizas los experimentos en la vida real”, comenta Ivica Slavkov, que comparte la primera autoría del artículo con Daniel Carrillo-Zapata. “Se podría decir que los robots son temperamentales. Rinden mejor por la mañana que por la noche, por ejemplo. Esto es porque las condiciones de iluminación son óptimas para su sistema de comunicación en ese momento”.
Todos estos detalles hicieron que el proyecto fuera un gran reto. La primera parte se realizó con simulaciones por ordenador y pasaron tres años hasta el enjambre de robots reales crearon la primera estructura. Las limitaciones de los robots también forzaron al equipo a que concibiera mecanismos sólidos e inteligentes para orquestar la generación de patrones del enjambre. Y eso hace que los enjambres de robots sean más parecidos a la biología real. La creación de formas a gran escala del enjambre es mucho más fiable que cada uno de los pequeños robots por separado: el conjunto es mayor que la suma de las partes.
Potencial para uso en el mundo real
Aunque la inspiración del proyecto surgió de la naturaleza, el objetivo final es crear enjambres de robots para usarlos en el mundo real. Imagina cientos o miles de pequeños robots creando formas o esculpiendo estructuras dinámicas en 3D que se adapten a cualquier tamaño y terreno. Por ejemplo, un puente de miles de robots después de un seísmo. “Como nos inspiramos en la creación de formas de la biología, en la que existe una auto organización y es resistente a los posibles fallos, los robots podrían seguir funcionando, aunque algunos de ellos estuviesen dañados.” dice Daniel Carrillo-Zapata. Aun así, hay un largo camino por recorrer antes que los enjambres de robots salgan del laboratorio.
James Sharpe (EMBL Barcelona) dirigió el proyecto Swarm-Organ, que se inició en el Centro de Regulación Genómica (CRG) cuando Sharpe era líder de grupo allí. Sabine Hauert (Universidad de Bristol) era la colaboradora veterana clave. Otros colaboradores fueron Fredrik Jansson (empleado actualmente en el Centrum Wiskunde & Informatica – CWI) y Jaap Kaandorp (Universidad de Amsterdam – UvA).
La investigación que dio pie a estos resultados recibió fondos del European Union Seventh Framework Programme (FP7) bajo el acuerdo de beca n° 601062 y el EPSRC Centre for Doctoral Training in Future Autonomous and Robotic Systems (FARSCOPE) en el Bristol Robotics Laboratory.
Creant estructures inspirades en la biològia amb centenars de petits robots
Científics de l’EMBL, del CRG i de la Universitat de Bristol programen un eixam de robots amb principis d’auto-organització per estudiar la formació de patrons
Centenars de petits robots poden treballar en equip per crear estructures semblants a les biològiques sense un pla mestre subjacent, sinó purament basats en comunicació amb robots veïns i moviment. Per aconseguir-ho, els investigadors han introduït principis biològics d’auto-organització a un eixam de robots. Science Robotics en publica els resultats el 19 de desembre.
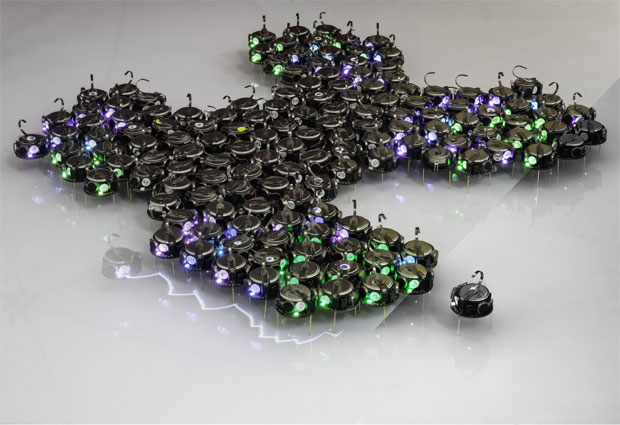
“Mostrem que és possible aplicar els conceptes naturals d’auto-organització a la tecnologia humana, com ara als robots,” diu James Sharpe, el cap de grup de l’EMBL Barcelona. “És fascinant, perquè la tecnologia és molt fràgil comparada amb la robustesa de la biologia. Si un component del motor d’un cotxe s’espatlla, en general acaba causant que el cotxe no funcioni. En canvi, quan un element d’un sistema natural falla, per exemple si una cèl·lula mor de sobte, no posa en perill el sistema sencer. Fins i tot la podria substituir una altra cèl·lula més endavant. Si poguéssim aconseguir la mateixa auto-organització i auto-reparació a la tecnologia, podríem fer-la molt més poderosa del que ja és.” Sharpe va liderar el projecte, que es va iniciar al Centre de Regulació Genòmica (CRG) juntament amb Sabine Hauert de la Universitat de Bristol.
La formació d’estructures tal com s’observa en l’eixam de robots. La llargada dels experiments és de tres hores de mitjana. Inspirat en la biologia, els robots emmagatzemen morfògens: molècules virtuals que transmeten informació del patró. Els colors indiquen la concentració de morfogen de cada robot xx: verd indica valors de morfogen alts, blau i lila indiquen valors més baixos i l’absència de color indica l’absència de morfogen en el robot. Cada robot emet la seva concentració de morfògen als robots en un radi de 10 centímetres. El patró de cercles d’alta concentració de morfogen dirigeix la relocalització de robots i el creixement de tentacles que emergeixen de l’eixam. https://youtu.be/bEm-fXkLw7g
Video: reimpresa amb permís de Slavkov, I., Zapata D. C. et al., Science Robotics (2018).
Les regles de Turing
L’única informació que l’equip ha introduït en els robots, que fan la mida d’una moneda aproximadament, són regles bàsiques sobre com interactuar amb els veïns. De fet, han programat els robots en l’eixam perquè es comportin com cèl·lules d’un teixit. Aquestes regles “genètiques” imiten el sistema que s’encarrega dels patrons de Turing que veiem a la natura, com ara la disposició dels dits en una mà o la taques d’un lleopard. D’aquesta manera, el projecte reuneix dues de les passions d’Alan Turing: la ciència computacional i la formació de patrons biològics.
Els robots es comuniquen mitjançant missatges transmesos per infrarojos amb els robots que es troben a dins d’un radi de 10 centímetres. Això fa que s’assemblin a les cèl·lules biològiques, perquè aquestes només poden comunicar-se directament amb altres cèl·lules que estiguin físicament a prop.
L’ eixam adopta diverses formes a mida que els robots es mouen de regions de baixa a alta concentració de morfogen, anomenats cercles de Turing. “És captivador observar l’eixam crear formes, sembla un procés orgànic. El que és fascinant és que no hi ha un pla mestre, aquestes formes emergeixen d’interaccions simples entre els robots. Aquesta és una diferència important amb estudis previs, on les formes sovint eren predefinides.“ explica Sabine Hauert.
Treballar amb grans eixams de robots
És impossible estudiar el comportament d’un eixam amb només un parell de robots. Per això, l’equip n’ha utilitzat almenys tres-cents en la majoria d’experiments. Treballar amb centenars de petits robots ja és un repte en si. L’equip d’investigadors van poder dur-ho a terme fent servir un mètode que facilita començar i parar els experiments o reprogramar tots els robots alhora fent servir llum. En total es van realitzar uns 20 experiments amb un gran nombre de robots, amb una durada aproximada de 3 hores per experiment.
Com en la biologia, un experiment sovint surt malament. Igual que les cèl·lules, els robots poden encallar o perdre l’eixam. “Són la mena de coses que no passen a les simulacions, sinó només en veritables experiments” diu Ivica Slavkov, l’autor principal de l’article junt amb Daniel Carrillo-Zapata.
Tots aquests detalls han fet que aquest projecte fos un gran repte. La primera part es va realitzar fent simulacions per ordinador i van passar tres anys fins que un eixam de robots reals va crear la primera estructura. Les limitacions dels robots, però, també van obligar l’equip a dissenyar mecanismes intel·ligents i robustos per orquestrar la formació de patrons de l’eixam. Inspirant-se en processos de creació de formes biològiques, l’equip va poder demostrar que les estructures creades pels robots podien adaptar-se a danys externs i auto-reparar-se. D’aquest manera, la formació d’estructures a gran escala de l’eixam és molt més robusta que cada un dels petits robots, és a dir, el conjunt és més que la suma de les parts.
Potencial per aplicacions en el món real
Encara que la inspiració del projecte sorgís de la natura, l’objectiu a llarg termini és utilitzar grans eixams de robots per aplicacions en el mon real. Imagina centenars o milers de robots diminuts creant estructures per explorar l’escenari d’una catàstrofe després d’un terratrèmol o un incendi, construint estructures dinàmiques 3D com per exemple un pont que pogués adaptar la seva llargada i forma per encaixar en diferents terreny o edificis. “Com ens vam inspirar en la formació de patrons biològics, que es caracteritza per l’auto-organització i robustesa, els robots podrien seguir funcionant, encara que alguns estiguessin danyats.” explica Daniel Carrillo-Zapata. Tot i així, hi ha un llarg camí per recórrer abans que els eixams de robot surtin del laboratori.
James Sharpe (EMBL Barcelona) va encapçalar el projecte Swarm-Organ, iniciat al Centre de Regulació Genòmica (CRG) quan Sharpe n’era un líder de grup. Sabine Hauert (Universitat de Bristol) va ser la col·laboradora sènior clau. També van col·laborar Fredrik Jansson (actualment treballa a Centrum Wiskunde & Informatica – CWI) i Jaap Kaandorp (Universitat d’Amsterdam – UvA).
The research leading to these results has received funding from the European Union Seventh Framework Programme (FP7) under grant agreement n° 601062, i el EPSRC Centre for Doctoral Training in Future Autonomous and Robotic Systems (FARSCOPE) al Bristol Robotics Laboratory.