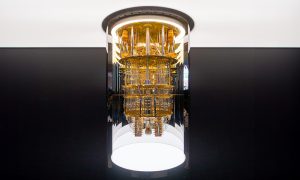
Read the latest Issue
How Christian Löw’s failed experiment led to an unexpected scientific journey
“I probably should have thrown those protein samples away,” says EMBL group leader Christian Löw. In 2009, one year into his postdoc, Löw was struggling to make any headway with his research into membrane proteins and set up yet another crystallisation trial in the vain hope of making that long awaited step forward. “I knew the prepared batch was contaminated with other proteins before we even started,” he says. “But we were under so much pressure to get results we went ahead anyway.” Predictably, the membrane protein he needed failed to crystallise. Yet Löw had unwittingly shone light on another group of proteins that help chains of amino acids – the building blocks of proteins – fold into a 3D structure.
The story starts over a decade ago when, as an undergraduate, Löw came across a protein known as SlyD. Proteins produced by the cell start off as long chains of amino acids, somewhat like a bead necklace. Only when these chains have been folded into a 3D shape can they do the jobs they are destined to do. For the most part, a chain of amino acids folds into a 3D shape by itself, but for certain arrangements it needs a helping hand. SlyD is a protein of two parts: a chaperone that grabs hold of the chain and pins it in place, so that an enzyme known as prolyl isomerase, can catalyse the changes needed. Although well studied, no one had been able to show just how SlyD does this – a problem that has long intrigued Löw, despite it not being the major focus of his research.
By following an unexpected lead, we’ve contributed significantly to the knowledge about protein folding
Years later, in temporary limbo while his PhD supervisor set up a new lab in Halle(Saale), Löw realised that crystallography could help him learn more about the atomic arrangements of SlyD. Shining X-rays on crystalline samples of the protein, he managed to determine the overall 3D structure of SlyD obtained from the bacterium Thermus thermophilus. Yet because protein crystals only capture one snapshot in time, he could not say much about how the protein actually functions. To do so he would have to capture the process at the precise moment the protein binds to the amino acid chain – and crystallise it in the act. “Chaperones such as SlyD only bind to their partners very fleetingly, often for only a few thousandths of a second – it didn’t seem possible,” Löw explains.
As he started his postdoc, SlyD remained a side project as he wrapped his mind around the challenge of studying membrane proteins. “Membrane proteins are the gateways to the cell and understanding how they let molecules in and out is crucial for working out how we might shuttle drugs into the cell, for example,” Löw explains. “It’s hard enough to crystallise proteins at the best of times, but membrane proteins are particularly difficult because they have to be extracted from the cell membrane before they can be crystallised. At the time there was a lot of frustration and we certainly did a lot of things wrong, but that’s the membrane business!”
One day after yet another crystallisation trial, Löw was surprised to see a set of well-formed crystals under the microscope. He knew these were far too forthcoming to be membrane protein crystal. But curiosity got the better of him: he took them to the synchrotron. “That was actually the first time I had been on my own,” he recalls. “I completely fried the few crystals, but nevertheless the data were encouraging.” Follow up experiments showed that he had unwittingly managed to crystallise a close relative of SlyD, but this time from the bacterium E.coli. “Before we can crystallise a protein, we need to make lots of it,” he explains. “We do this by inserting the gene that codes for the protein into E.coli, tricking it into producing the protein we need. In our case this didn’t work and instead of membrane proteins, we managed to crystallise a contaminant from E.coli.”
Failure is an important part of science: Even if it’s not going as expected, there could be something interesting there
But even this SlyD did not bind long enough with the unfolded amino acid chains to be crystallised. Months later, frustrated with another dead end, Löw struck upon an idea. What if he combined the T.thermophilus SlyD with amino acid chains identified for the E.coli SlyD? “Thermus thermophilus loves extremely hot temperatures, while E.coli prefers lower temperatures,” he explains. “Typically the higher the temperature the weaker the binding, so I wondered if combining them in this way would hold it together longer so that we could finally have a long enough time window to crystallise the moment the protein binds to the amino acid chain. And I was right!” he says with a smile.
Ten years of trials, tears and tribulations has now resulted in the publication of a data-rich paper in BMC Biology, including structural information and a proposed catalytic mechanism. “By following an unexpected lead, we’ve contributed significantly to the knowledge about protein folding,” says Löw. And as for his membrane research? “We are a lot better at it now than we were eight years ago! Although it is still a challenging field, we have many more tools and methods at our disposal. Failure is such an important part of science: most important is to be able to look at your experiments and, even if it’s not going as expected, there could be something interesting there. That is what makes a scientist a scientist. That is science.”
Looking for past print editions of EMBLetc.? Browse our archive, going back 20 years.
EMBLetc. archive