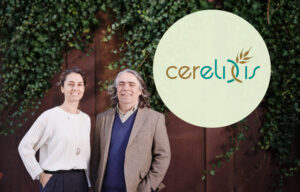
Cell control in a flash
Flick the switch, and illumination follows. The comical image of a light bulb pinging on when a brainwave occurs became remarkably prescient just a few years ago when suddenly, out of the dark, a brand new technique for controlling brain activity lit up the scene.
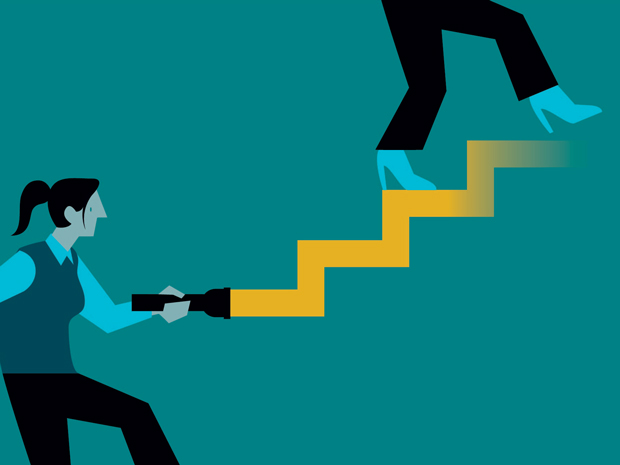
By Adam Rutherford
Emerging in 2003, optogenetics was developed by several scientists across the globe, including Ernst Bamberg, Ed Boyden, Karl Deisseroth, Peter Hegemann, Gero Miesenböck, and Georg Nagel. The aim was to elicit control over ever-smaller neural pathways, trying to unpick the circuits of thought. But the simplicity of the idea betrays an impressive and imaginative technical feat. The principle is straightforward: a light-sensitive protein is incorporated into a specific population of cells, and a laser tuned to that protein’s specific firing frequency is used to activate the cells with millisecond precision. The timing is crucial, as it mirrors the physiological response time, and the light triggers the neuron’s action potential.
Standard techniques of genetic modification, such as the cre/lox system and lentiviral vectors, are used to target the gene encoding a phototransduction protein – often a microbial opsin – to specific neuronal cells. But the more fiddly aspect of this technique is that this has to be done in such a way that normal function is not upset. The use of optogenetics in the past decade has blossomed, and uncovered all sorts of revelations about brain function – for example, the circuits that modulate fear and the conditioning of cells involved in cocaine dependency. Speculation has been rife for some time that sooner rather than later, this is a tool that will bag a few Nobel Prizes.
we are very excited about adding this new technique to our research arsenal
In touch
Paul Heppenstall, a group leader at EMBL Monterotondo, has now adapted the system for use outside the darkness of the brain. “It was the ability to look through the microscope and image neurons under the skin that got me excited,” he explains. Heppenstall’s team work on our perception of touch and pain, which means their targets are the cells at the periphery of the nervous system – neurons that react to mechanical or chemical stimuli, touch, heat and pressure. The specific populations for each of these are poorly understood, and not easy to disentangle.
It was the ability to image neurons under the skin that got me excited
“Traditionally we’ve used electrophysiology of single cells, which is tedious and slow,” says Heppenstall. “It takes several months to put the data together, and at the end, you might see something. Or you might not.”
Their new technique involves identifying a cell-specific marker, a gene that is active in a discreet population of skin neurons. They do this by scanning the literature, making educated guesses and also through a bit of luck. Once they find such a gene, they attach an optogenetic control switch onto it using the cre/lox system. Then they can activate the cells by shining blue light on a patch of skin and monitor the behaviour this evokes in mice, such as withdrawal for pain-sensing neurons and scratching for itch receptors. As a complementary experiment, they also use the same system to ablate specific populations of cells through a photosensitive dye. Once they’ve ablated the cell, a stimulus can be applied and changes in behaviour studied.
Shaping up
Optogenetics offers cell-specific and microsecond precision. What’s odd is that this powerful technique has always been about brain cells. Even in the 1970s, Francis Crick pondered light-controlled cells, but only for neurons.
I knew it would be great if it worked, but to tell you the truth, I was quite sceptical
At EMBL Heidelberg, group leader Stefano De Renzis is set to break that stranglehold. “The biggest surprise for us was that it’s a technique that works so well for studying Drosophila morphogenesis,” he explains. It was De Renzis’ graduate student Giorgia Guglielmi who first suggested co-opting optogenetics from neuroscience to scrutinise basic fruit fly development. “I knew it would be great if it worked, but to tell you the truth, I was quite sceptical. Giorgia who had just started her PhD in my lab was immediately enthusiastic to try something new.”

Their work is centred on how proteins and other elements of the cell membrane regulate the shape of cells during Drosophila embryogenesis. This is crucial to overall tissue patterning as cells mature and take on specific shapes and sizes. Differentiation of individual cells affects their neighbours and ultimately the whole embryo. But these changes are highly temporal and spatial, and addressing the underlying mechanisms is a question that has been hampered by an inability to command the whens and wheres of gene expression. And there’s no action potential to trigger.
Knocking down
“We were always looking for ways of controlling specific cells during development – to knock-down protein activity, but in a very temporal and spatial way,” De Renzis says. “In the past we’ve used knock-outs, and temperature-sensitive mutants, but it takes time for the clones to grow, which complicates the interpretation of results. With optogenetics we can do it in a fraction of the time, enabling us to look at immediate cell and tissue responses.”
In some ways, this seems a simpler proposition than eliciting complex behavioural changes in mammals, as has been the mainstay of optogenetics so far. The De Renzis team is looking at actin and membrane trafficking, and how they affect patterning and cell shape. They are quizzing the way the embryo invaginates – a process of folding in on itself to internalise mesodermal cells that run the length of the fruit fly embryo. This is regulated by molecules on the plasma membrane, and by simply turning these off using light in only a small population of cells, the effect is global. The targeted cells fail to contract, and the knock-on effect is the arrest of invagination across the whole embryo.
This first foray into using optogenetics in developmental biology is now submitted for publication and no doubt the technique is set to become a versatile tool in the future. “To control cellular activity experimentally has not been possible with conventional genetics so far,” De Renzis says, “at least not at very precise resolution. Now, we are very excited about adding this new technique to our research arsenal and we look forward to sharing it with other labs interested in shedding light on the cellular and molecular mechanisms driving the development of organisms.”