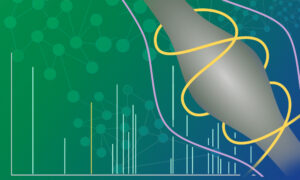
Read the latest Issue
EMBL alumna Mariëlle van Kooten is part of a research community working to beat heart disease
By Mariëlle van Kooten, Markus Grosch, and Julia Kornienko
With scientific cliffhanger endings to each Zoom catch-up, a massive community of scientists edges closer to therapeutic strategies for heart disease. Centre of operations: Heidelberg, Germany.
Our object of study, the human heart, contains an estimated three billion cardiomyocytes, or heart muscle cells, which undergo relentless mechanical contractions throughout life. Cardiomyocytes have to adapt their metabolism to ensure continuous mechanical activity, but it’s a trade-off: these changes in the cell’s chemistry also lead to age-related decline in cardiac performance. More importantly, mammalian cardiomyocytes have a limited ability to regenerate. In a literal sense, the heart does not heal.
Heart disease is the leading cause of death worldwide, and donor replacement of the heart is the sole option available in life-threatening conditions. We don’t yet understand the cellular mechanisms behind the healthy, the maturing, and the diseased heart well enough to prevent, repair, or regenerate heart tissue.
The development of heart disease is a complex process that involves many factors, requiring unprecedented research breadth and detail. Take, for example, dilated cardiomyopathy (DCM) – a disease in which the heart muscle becomes larger and weaker, and is less able to pump blood. DCM may be caused by environmental factors, but familial DCM has a genetic origin. Together, DCM and familial DCM have an estimated prevalence of 1 in 250 people worldwide. A subset of familial DCM patients carry mutations in the gene coding for a protein called RBM20. The mutations may cause RBM20 to form granules. Because this process has similarities to the neurodegenerative disease amyotrophic lateral sclerosis, the granules are expected to play a much wider role in heart disease. “The mechanistic parallels with neurodegenerative disease are compelling and fascinating, opening a new avenue of research that cuts across both fields,” says Jay Schneider, a cardiologist at the Mayo Clinic.
This is just one example that demonstrates the need for in-depth, comprehensive study of heart disease. It’s a process that requires broad expertise – and many minds. “We’re collaborating with biologists, engineers, data scientists, and clinicians across the globe to get to the bottom of RBM20 DCM,” says EMBL Senior Scientist Lars Steinmetz. “Our genome is not our destiny. Understanding how RBM20 mutations cause degeneration of the heart will help us identify biomarkers to monitor disease progression and even design new therapies so these patients can live longer, healthier lives, and ideally never develop DCM in the first place.”
It’s not easy to get donor material, so one way to study heart tissue is by using cells with the elaborate name of human induced pluripotent stem cell-derived cardiomyocytes (hiPSC-CMs). These are created by taking cells from another part of the body and genetically reprogramming them to become heart cells. After reprogramming, the cells undergo a transition in which they assemble the central structures responsible for creating mechanical force in the heart, known as sarcomeres. These are interconnected molecular structures that are responsible for – and help to synchronise – the contractions of each cell. They create your heartbeat. Observing how gene activity is translated into functional molecular machines is absolutely stunning: hiPSC-CMs beat in a Petri dish.
In addition to the wide-ranging causes of heart disease and the limited availability of sample material, researchers meet with a number of confounding hurdles in experimental studies of the heart. For one, cardiomyocytes form a highly diverse cell population. Although each cell carries the same genome, gene expression depends, among other factors, on a cell’s location. For example, the gene TTN gives rise to two distinct gene products, and the ratio between these products differs in the cells of the heart’s upper and lower chambers, known as the atria and ventricles. During the process of converting information from DNA into proteins, instructions from genes like TTN can be cut and spliced together in different ways, giving rise to different gene products, called isoforms. In the case of TTN, the expression ratio between the isoforms allows the ventricles to contract and recoil rapidly.
The diversity of cell types means that any study should be set up in a way that distinguishes individual cells. However, cardiomyocytes can reach immense sizes – too large for typical single-cell measurement platforms. In addition, cardiomyocyte cell biology involves several long isoforms like those arising from TTN, and making quantitative measurements of these is a painstaking and difficult process. Indeed, another gene that plays an important role in heart cells, DMD, is one of the largest genes found in humans, containing around 2.3 million letters of the DNA code.
A further hurdle appears after making the transition from the Petri dish to studies in living organisms, because there are significant differences in heart disease processes between humans and organisms that are used to study these processes in the lab, such as zebrafish and mice. This hinders the translation of experimental results, and means that findings from different model organisms have to be pieced together to form a logical storyline, in which not all puzzle pieces will fit. To overcome these hurdles, we need novel experimental and analytical tools.
The interdisciplinary expertise of our consortium’s members and their willingness to work together help to drive our research, leading to faster problem solving and accelerating the process of identifying and testing drug candidates for treating heart disease. Dr. Benjamin Meder, a physician scientist at Heidelberg University Hospital, likens it to a symphony orchestra: “Heart disease is a complex composition. But, by working in harmony, combining insights from genomics, molecular biology, artificial intelligence, and medicine, our research can be life changing.”
Looking for past print editions of EMBLetc.? Browse our archive, going back 20 years.
EMBLetc. archive