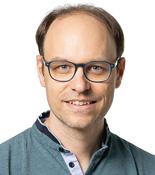
Robert Prevedel
Group Leader
robert.prevedel [at] embl.de
ORCID: 0000-0003-3366-4703
EditAdvanced optical techniques for deep tissue microscopy
Group Leader
robert.prevedel [at] embl.de
ORCID: 0000-0003-3366-4703
EditLocated in the Cell Biology and Biophysics Unit, EMBL Heidelberg. Affiliated with the Developmental Biology Unit, EMBL Heidelberg, and the Epigenetics and Neurobiology Unit, EMBL Rome.
Light microscopy has revolutionised our understanding in many areas of biology, and over the years tremendous progress has been achieved by imaging cellular and subcellular processes in transparent, fixed, or thin sample preparations. Yet in order to obtain a complete understanding of biological processes, in vivo studies inside thick, three-dimensional living tissues are often required. However, when light interacts with thick biological tissue, the process of light scattering leads to low-resolution, ‘blurry’ images and an effective loss of excitation power with increasing imaging depth. This has severely limited the biomedical usefulness of light microscopy in studying, for example, cultured cells in vitro or superficial layers of tissue in vivo. New approaches and tools are thus required to non-invasively image biological functions at depth inside living tissue with sufficient resolution, speed and contrast.
In the past, we have developed novel optical techniques for high-speed imaging, with a particular focus on functional imaging in the neurosciences. Among other things, we have put forward a two-photon microscopy technique based on light-sculpting, which has enabled the first whole-brain calcium imaging in C. elegans. In other work, we have established light-field deconvolution microscopy, an elegant approach to performing volumetric imaging, which achieves unprecedented acquisition speeds while requiring no mechanical scanning. Recently, we extended our imaging methods to the scattering tissue domain and demonstrated fast volumetric calcium imaging across the majority of a cortical column in the mouse. Together with our collaborators, we apply our methods to study neuronal activity and cellular dynamics in a range of model organisms such as zebrafish larvae or behaving mice (see Fig. 1). Another focus of the group is to develop new optical techniques based on Brillouin scattering to ‘image’ mechanical properties of living tissues in a non-contact fashion and with diffraction-limited resolution in 3D (see Fig. 2).
The future focus of the group is to push the frontiers of deep tissue microscopy in terms of imaging depths and resolution by developing advanced and innovative optical imaging techniques. To do so we actively work in diverse fields such as multi-photon microscopy, active wave-front shaping, photo-acoustics as well as Brillouin spectroscopy. In particular, we intend to combine these into multi-modal imaging systems that allow the study of cellular processes and dynamics at depths thus far inaccessible by conventional microscopy. Our multidisciplinary team comprises physicists, engineers, computer scientists and biologists, and we engage in close collaboration with fellow groups at EMBL in the fields of cell and developmental biology as well as neuroscience.