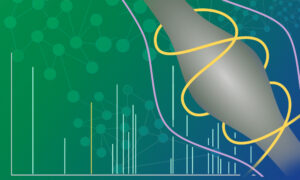
Read the latest Issue
Exploring what it would take to regrow a lost limb, and what we might learn along the way
If you were to attempt to regrow an arm, there would be two ways to go about it, says James Sharpe. You could either coax the body into regenerating the limb, or you could produce an arm in the lab and graft it on. Of course, neither option is as straightforward as it may sound.
Sharpe is Coordinator of the Systems Biology Programme at the Centre for Genomic Regulation (CRG) in Barcelona, where his lab tries to understand precisely how limbs are formed. “We know quite a bit more about limb development than about some other systems or organs,” says Sharpe. Unlike the heart or the brain, limbs are not essential for life. From a scientist’s standpoint, this makes them ideal: while they are still just tiny buds of tissue, barely 1mm across, you can interfere with them without destroying their ‘life-support system’ – the rest of the embryo. So as early as the 1940s scientists were opening chicken eggs and studying how a chick’s wing is formed. Like us, chickens are vertebrates, so a lot of what was learnt in those early studies probably holds true for humans. Over the years, thanks to advances in genetics and microscopy, our knowledge of how limbs form has grown in leaps and bounds.
making sense is not the same thing as being true
Scientists now know many of the molecules that sculpt a mass of embryonic cells into a functioning arm, hand and fingers. They have uncovered gradients, where a particular molecule is found in high concentrations near the body, and diffuses out so that the further away from the body a cell is, the less it is exposed to that molecule. Like markers by the side of the road, these gradients tell cells how far along the arm they are, so that cells know when to stop growing and start making a hand. In the hand, Sharpe and others have found an interplay of ‘activating’ and ‘repressing’ molecules that seems to define the strips of cells that will become fingers, and those that will be chiselled away to create the spaces in between.
“We have a lot of information, but we’re coming to the conclusion that a lot of our stories about how this system works, based on that knowledge, are probably dramatically simplistic,” Sharpe warns. “They make sense, but making sense is not the same thing as being true.” Nevertheless, Sharpe is confident that the truth – however convoluted – is within reach. “I think we’re in the perfect situation that maybe 10 years from now we will have a really confident view about the complexity of all this,” he says.
Nadia Rosenthal is similarly excited about the prospects of regenerative medicine. “I think the next decade is going to be very exciting,” she says with a smile. “Now that the field is really thinking about what a tissue needs to regenerate itself, we’re going to come up with ingenious ways to make that happen.”
We know that salamanders can do this, so the question is why can’t we?
Rosenthal was Head of EMBL’s site in Monterotondo from 2001 to 2012, Scientific Head of EMBL Australia from 2010-2015 and is now Scientific Director at The Jackson Laboratory for Mammalian Genetics, USA, and Chair in Cardiovascular Science at Imperial College London. Her lab investigates how muscles regenerate. Rosenthal is interested in teasing out the differences and similarities between the skeletal muscles that you show off when you flex your arm and the heart muscle that beats in your chest. As anyone who’s pulled a muscle can attest, skeletal muscle can regenerate. The heart, on the other hand, has much less regenerative capacity. Rosenthal and her labs use mice as a proxy for humans, but they also look at animals that can grow back an arm: salamanders.
“We know that salamanders and other animals can do this, so the question is why can’t we? What are the biological impediments for this in mammals?,” she ponders. One difference is that injured salamanders bear no scars. In mammals like us, injury triggers a response by the immune system. If the resulting inflammation doesn’t subside in time, cells in that tissue die off, and are replaced with scar. As scar tissue isn’t functional muscle, bone or skin, it becomes a barrier, preventing the arm from growing back. This scarring can make it very hard for a patient to fully recover from a heart attack or an injury to the spinal column. Rosenthal and colleagues have found that in salamanders, the chemical signals that immune cells exchange amongst themselves and with the injured tissue are essential for the animal to regenerate parts of its body. But even if we were able to learn the salamander’s ‘tricks’ and somehow co-opt them to prevent or remove scarring in a human patient, that wouldn’t be enough for the person’s arm to grow back. We’d have to somehow jumpstart growth.
Adding stem cells or other cells with a propensity to build specific types of tissue would be a start, but those cells would require instructions. These could be the ‘roadsign’ and patterning molecules that shape the embryo’s arm. But other factors could also be at play. Scientists at MIT, for instance, have recently shown that differences in cells’ ability to conduct electricity could also help cells gauge their position. “This would be a much more nuanced way to control things than chemical gradients,” says Rosenthal. “And if it turns out to be true, it makes the thought of zapping a tissue to jumpstart growth less sci-fi than you’d think!”
In any case, both Rosenthal and Sharpe point out that, valuable as the insights from developmental biology are, regrowing an adult arm poses its own challenges. “When you regrow a limb, you have to regrow it out of an organism that has a fully developed immune system. It also has a fully developed vasculature, nervous system… and it’s also much bigger than when the limb was beginning to grow in the embryo. So it’s just naïve to think that it’ll boil down to a simple recapitulation of development,” Rosenthal cautions.
To understand how regeneration overcomes the hurdles of adulthood, scientists can probe salamanders and other ‘regenerators’. But there are also clues closer to home. Humans may not be able to grow back an arm or a leg, but some of our organs do have remarkable regenerative capacity. “You can basically cut away two thirds of the liver and within two weeks the liver mass has been restored,” says Helmuth Gehart, a postdoctoral fellow in Hans Clevers’ lab at the Hubrecht Institute in the Netherlands. The liver has several lobes; if a lobe is removed, the remaining lobes grow until they are able to take over all functions of the lost liver tissue. Looking at what drives this regeneration – and what constrains it – could give clues of how to coax other tissues or organs into rebuilding themselves. For the most part, scientists like Gehart, Rosenthal and Sharpe are still at the clue-gathering stage.
Even if one day we manage to master regeneration, growth and development to the point that we can think of making a person’s arm grow back, salamander-style, there could still be a practical hurdle. “One would imagine that growing back a whole arm would be quite slow,” Sharpe ponders. “What would you do during that time? Would you have to keep the patient in hospital, in isolation, for months?”
Considering the challenges in getting a patient’s arm to regenerate, would it be easier to grow them an arm in the lab instead? This is one of the approaches that Gehart uses to try to understand how the liver rebuilds itself: getting it to do so – albeit at a much smaller scale – in a lab dish. He is among a growing number of scientists turning to organoids: essentially, balls of cells that act like miniaturised versions of an organ. Gehart is keen to stress that he is not in fact growing livers in a dish. “These organoids have different cell types, and they have all the functions of the organ – they do what the organ normally does – but they have no blood vessels, no nerves, no connective tissue,” he explains. Because they have no support structures, cells in these organoids lack important cues that would allow them to arrange themselves into whole organs. So the largest organoids scientists can produce at present are only one or two millimetres in diameter. Can the approach ever get to the stage where we can build a whole arm?
In order to grow an arm in a lab, you’d have to be able to pump blood through it, and, as with getting a person’s arm to regenerate, it would probably help if you really knew the drivers of arm development. But aside from that, says Sharpe, you’d face a spatial challenge. “An arm has a certain proportion of muscle cells, bone, tendon, skin, etc. But if you just mixed the right number of cells together in a flask at random, most of the possible configurations are not an arm! So an exciting challenge for us in my lab,” he says, “is how do tissues organise themselves, geometrically?” In an effort to find out, Sharpe’s lab in Barcelona uses a combination of experiments, imaging and computer models. “These systems are beautifully, fascinatingly complicated, so computer modelling is absolutely essential, because humans are not very good at thinking of thousands of cells, in 3D space, changing over time.”
“I don’t know of anyone who is trying to grow an arm in a lab,” says Sharpe. Considering all the biological challenges, and given the advances in prosthetics, it’s possible that by the time we are in a position to regrow a ‘natural’ arm, artificial ones may have supplanted the ‘real thing’. Or the two fields may merge, offering future patients bionic arms. Ethical considerations will also come into play, not least in the context of how we view disability.
For now, progress will likely come in incremental steps. Scientists are beginning to be able to build relatively simple tissues like skin, for example. And researchers have recently been able to grow heart cells that can beat, by seeding them onto a flexible scaffold, which allows the cells to stretch and create the beating movement they’d have in the heart.
Building from that, says Sharpe, it seems quite plausible that as the field matures, it will become possible to build more complex arrangements – perhaps combinations of bone and muscle, for instance – that could be transplanted back into the body. “Ironically, these ‘little steps’ may even be more beneficial than being able to build a whole arm,” he points out, “because a lot of the damage that humans have to put up with isn’t as dramatic as losing an arm; it’s parts of an organ dying off or getting damaged for all kinds of reasons.”
Some of those baby steps are already helping patients. Doctors can already treat burn victims, for instance, with skin grafts grown from the patient’s own cells. These grafts are not yet perfect. They don’t have hairs or sweat glands, although promising work in mice indicates that may come soon. And even these imperfect skin grafts can serve as a scaffold for the patient’s own skin to grow back on.
Clinical trials are testing similar approaches for patching patients’ knees with cartilage grown from their own cells. And just this year, two clinical trials reported encouraging results of patients who were able to see again after receiving transplants of cornea grown in the lab – either from their own cells or from cells of donors.
As scientists in these fields look to other areas of engineering for inspiration, entirely new approaches can emerge. “I just spoke to someone at a conference who has developed microparticles that could be delivered to the right place in the body and release factors that would stimulate regeneration,” says Rosenthal. “You could imagine that that sort of control would allow for a lot more refinement in the way we deliver drugs, too.”
I absolutely don’t think it’s complete science fiction
Not all promises will be fulfilled, but they’ll all bring new insights, says Rosenthal: “We learn as much from what doesn’t work as we do from what does.” And some of that knowledge can also be harnessed to stop unwanted growth. “Many of the aspects that we study in regenerative medicine have a lot of applications in cancer, of all things,” says Rosenthal. Many of the parameters that she and others aim to stimulate for regeneration need to be reined in to treat the uncontrolled growth that is cancer.
So will we ever be able to regrow an arm? Going back to Sharpe’s options of getting the body to regrow or creating an arm in the lab, Sharpe, Rosenthal and Gehart all agree that if we ever find a solution, it’s more likely to be something in between: growing components in the lab, and grafting them on to the body with the right stimulants to form a functional arm. And they all predict it won’t happen any time soon. “There’s not going to be a eureka moment when someone grows back the legs on a Paralympic athlete,” says Rosenthal, “I think it’s going to be much more gradual.”
“I absolutely don’t think it’s complete science fiction,” says Gehart, “but we clearly need a better understanding.” As that understanding grows, more and more people may receive a helping hand – at least figuratively speaking.
Since September 2016, EMBL and the Hubrecht Institute in Utrecht, the Netherlands, have an institutional partnership dedicated to research in stem cell and tissue biology.
Researchers in this partnership investigate how human tissues and organs develop and are organised, advancing our understanding of a wide range of diseases, including heart degeneration, Alzheimer’s, diabetes and tumours.
Find out more
EMBL and the Centre for Genomic Regulation (CRG) in Barcelona, Spain, have an institutional partnership focused on systems biology since 2006. The partnership aims to address issues in the field of systems biology, and further our understanding of key aspects of biology that are relevant to human health. It brings together experts from a variety of scientific disciplines – from biochemistry and genomics to computational biology – to study how molecular systems and cells function as a whole.
More information
Looking for past print editions of EMBLetc.? Browse our archive, going back 20 years.
EMBLetc. archive