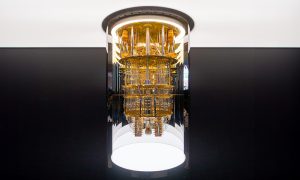
Read the latest Issue
EMBL conference explores how genomics can shed further light on what we know about cancer
By Nivedita Mukherjee, PhD student, Computational Cancer Genomics Lab, National Center for Biological Sciences, Bangalore, India
Recently, more than 300 scientists convened at EMBL amidst the vibrant fall foliage in Heidelberg, Germany, and almost 100 more joined the hybrid conference online. Their shared goal: to unravel the centuries-old puzzle of cancer, viewed through the modern lens of genomics. Here are just six highlights from the three-day discourse:
Newly born cells are often genetically different from their parent cells in subtle ways – these changes are called mutations. Studying exactly where mutations appear in the DNA sequence can tell us a lot about how they arise. Marketa Tomkova from the University of Oxford, UK, used a specialised sequencing technology called PER-seq to discover that replication errors – mistakes made by cells while copying their DNA – actually contribute to a much larger fraction of a particular class of mutations than previously believed.
According to Sabarinathan Radhakrishnan from the National Center for Biological Sciences, India, replication errors are also responsible for the extremely high mutation rates in certain DNA regions in cancer cells, specifically regions bound by the CTCF/cohesin complex – a protein complex crucial for the structural organisation of the DNA within cell nuclei.
Whether stemming from replication errors or not, mutations aren’t always a problem since cells have mechanisms in place to fix them. But if the proteins responsible for DNA repair themselves get mutated, it can unleash a catastrophic cascade of mutations throughout the genome, resulting in genomic instability. This phenomenon, that cancer cells are infamous for, can pose interesting questions for researchers.
One such gene is XPD, associated with the extreme sensitivity of Xeroderma Pigmentosum patients to UV-induced skin damage. Jayne Barbour at The University of Hong Kong found that bladder cancer patients with XPD mutations show a very distinct distribution of mutations across the genome, including increased mutation rates at the CTCF-cohesin binding sites.
In discussions regarding genome instability, the DNA repair gene that always takes centre stage is TP53. Known as the ‘guardian of the genome’, it also happens to be the most frequently mutated gene in cancer. Aurélie Ernst from the German Cancer Research Center (DKFZ) discussed how TP53 mutations can lead to spontaneous ‘chromothripsis’ – a complex mutational event where the genome gets shattered into pieces and restitched randomly – much like a puzzle put together in a hurry that now shows a morphed version of the hidden picture.
Isidro Cortes Ciriano from EMBL-EBI also discussed the link between TP53 and chromothripsis, specifically one that’s initiated through chromosomal rearrangements in osteosarcoma patients.
Approximately 45% of the human genome comprises transposons or ‘jumping genes’. If active, these unique genetic elements can replicate themselves and insert elsewhere in the genome. While cells have evolved mechanisms to regulate the activity of transposons and safeguard the genome’s integrity, they are not failsafe.
Young Seok Ju from the Korea Advanced Institute of Science and Technology, Republic of Korea, found several retrotransposons, a class of transposons, active in the lining of the large intestine, with ‘jumping’ events (and hence the risk of mutation) increasing in frequency with age and upon the onset of cancer.
E. Alice Lee from Harvard University in the United States studied how these retrotransposition events impact the genome and contribute to cancer. They observed that active retrotransposons increase genomic instability leading to chromosomal rearrangements including chromothripsis events, and also often insert themselves in the vicinity of cancer-relevant genes. By doing so, they can activate cancer-promoting genes or deactivate cancer-suppressing genes.
Apart from spontaneous events, mutations also result from exposure to external agents, called mutagens, such as certain drugs or chemicals. Many chemotherapeutic drugs can induce mutations in DNA. This makes chemotherapy a necessary evil; it kills cancer cells, while holding the potential to further mutate them into becoming chemotherapy-resistant or even to trigger independent secondary tumours.
Since different mutagens leave behind different patterns of mutations on the genome, these can be viewed as signatures and used to trace back the initial exposures. By exploiting this principle, Jurrian de Kanter from the Princess Máxima Center for Pediatric Oncology and Oncode Institute in The Netherlands found high numbers of platinum treatment induced mutations that made cells cancerous in paediatric tumours. Additionally, he tracked these cancer cells through time and noticed how they took less time to become chemotherapy-resistant if the TP53 gene was already mutated.
On a similar track, at KU Leuven in Belgium, Ilana Struys sequenced cord blood DNA in pregnant mothers undergoing chemotherapy to find evidence for treatment-induced mutations in the babies’ stem cells. The talk gave rise to discussion on optimising treatment schedules for mothers undergoing chemotherapy to minimise adverse effects on the developing foetus.
Within the vastness of the genome, only some mutations contribute to transforming a normal cell into a cancer cell. This subset of mutations present in cancer cells comprise what are known as cancer driver mutations, and the genes carrying them are called cancer driver genes.
One way we can identify driver mutations is to look at how frequently they are found in cancers. Mutations that pop up again and again in multiple cancer patients are likely to be important for that type of cancer. However, this makes it difficult to identify relatively rare cancer driver mutations. In an effort to study these rare mutations and identify which can be used as drug targets, Carla Schmidt from the University of Freiburg and German Cancer Consortium (DKTK), Germany, selected the FGFR genes – which encode a protein that acts as a growth factor receptor – as a suitable candidate. These genes encode proteins that are involved in signalling cells to grow and are often dysregulated in cancer cells. She created over 10 thousand versions of these genes, each with a unique mutation, and found several hundred mutations that make cells more or less sensitive to FGFR inhibitor drugs.
Scaling up this effort, Núria López-Bigas from Institute for Research in Biomedicine Barcelona, Spain, carried out a similar experiment but in silico. Using a machine learning model called BoostDM, her lab characterised all possible mutations in several cancer driver genes in different tumour types, selecting out ones most likely to be cancer driver mutations. As part of the Cancer Grand Challenges programme, her team now uses such methods to identify driver mutations in normal cells to understand how that relates to risk of developing cancer.
With cells continually accumulating new mutations, a tumour becomes a milieu of genetically distinct cellular clones that evolve, akin to animal populations in an ecosystem. However, unlike animals, tumours don’t leave fossils that we can use to trace their evolutionary histories. Hence, the problem of studying tumour evolution asks for creative solutions.
One approach to detangling clonal populations in a tumour uses computational tools on the DNA sequences of individual cancer cells. Sam Behjati from Wellcome Sanger Institute, UK, has employed such techniques to crack the mystery of childhood cancers and their resemblance to developmental disorders.
For a clearer view of the clonal structure, Hugo Snippert from University Medical Center Utrecht, The Netherlands, has been comparing sequences from normal, precancerous, and cancerous tissues of the same tumour, uncovering genetic triggers of invasiveness in sarcomas. Snippert also figured out a way to couple live-cell imaging with DNA sequencing of single cells that he applies to 3D organoids – lab-grown tumours derived from patient cancer cells.
Patrick Tan from Duke-NUS Medical School, Singapore, studies tumour evolution by sequencing biopsies at different stages of gastric cancer development. Instigated by infection of the H. pylori bacteria, gastric cancer passes through an intermediate premalignant stage, but not all cases of such premalignancy progress to stomach cancer. Tan found that cases that advance to malignancy exhibit specific driver gene mutations and a higher, though less diverse, bacterial load.
Tackling a similar problem at the University of Cambridge in the UK, Rebeca Fitzgerald identified mutation signatures and driver gene alterations associated with the malignant transformation of oesophageal cancer from its precursor. She also introduced a non-invasive alternative to endoscopic examination for screening patients at risk called the Cytosponge, a pill that expands into a sponge when swallowed and can be pulled out while gently scraping cells from the epithelial lining.
In between grabbing break-time beverages and rushing for talks, I was struck by just how diverse the problem of cancer is. It can’t be reduced to generalisable principles and unified cures, thus requiring an army of scientists to read the unique stories each tumour has to tell. The above reports only on a miniscule of ongoing efforts, but I tip my hat to everyone who has joined the force.
Want to learn more about cancer genomics? EMBL will hold a ‘Cancer genomics and transcriptomics’ virtual course 24-28 June 2024 with space for 35 participants. Find out more about the course and how to apply here. Applications close on 31 March 2024.
Looking for past print editions of EMBLetc.? Browse our archive, going back 20 years.
EMBLetc. archive