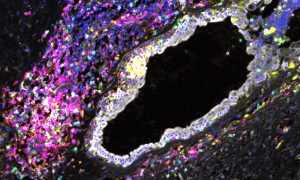
Read the latest Issue
Studies of radiocarbon are helping EMBL researchers shine light on how neurons stay stable yet adaptable.
On 30 October 1961, a mushroom cloud more than seven times the height of Mount Everest rolled skywards over Novaya Zemlya, an archipelago in the Barents Sea. It was the signature of the Soviet Union’s 50 megaton Tsar Bomba, the biggest atomic bomb ever detonated. Its physical shockwave cracked windows 900km away, but its political impact was even greater and helped to trigger an international ban on above-ground nuclear bomb tests. Now, more than half a century later, scientists at EMBL are finding a positive aspect to this dark era of the Cold War: radiocarbon, a harmless component of the fallout from these tests, is providing a window on the workings of the human brain.
Tracing the behaviour of radiocarbon is helping EMBL Heidelberg group leader Kyung-Min Noh and her colleagues in the US to understand how our neurons, the longest-lived cells in our bodies, stay both stable and yet flexible enough to let us learn, remember and think throughout our lives. What’s more, they hope the work will provide new insights into brain development defects such as autism, and possibly also other conditions such as Alzheimer’s disease.
Carbon is the backbone element of all the biological molecules in our bodies. Almost all the carbon in the world comes in a ‘regular’ form called carbon-12 that has a particular weight. Radiocarbon is a slightly heavier, mildly radioactive form that occurs naturally in tiny amounts. The above-ground atomic tests pumped a pulse of man-made radiocarbon far in excess of these natural levels into the atmosphere between 1945 and 1963. This made its way into the food chain all over the world, meaning that people who lived through this era incorporated more radiocarbon than normal into their bodies. Once atmospheric levels had dropped back to regular levels, their bodies gradually replaced most of this radiocarbon with regular carbon, as their cells naturally renewed themselves over time.
But different parts of the body renew at different rates, so scientists can work out which cells are replaced and how often by measuring the amount of radiocarbon in the various tissues of people who lived through the atomic-test age. Ten years ago, a team of scientists in Sweden and the US used this technique to show that neurons in some parts of the brain renew themselves throughout life whereas others stop at birth and are as old as the individual to whom they belong. Now Noh and her colleagues have adapted this approach to tackle one of the big mysteries of neurobiology: how do these enduring neurons remain stable yet adaptable?
Part of the answer lies in the DNA of the neurons. This DNA contains genes, which instruct the neuron to make tiny molecular machines called proteins that enable the neuron to function. Even though almost every cell in our bodies contains the same set of genes, each cell type uses a different subset of these genes to develop its specialist function. This means that the cell needs to keep certain genes active and others inactive.
One way cells do this is by changing the way the DNA is packaged inside cells. Rather than floating around in a tangled mess, DNA is wound around proteins called histones, rather like thread coiled around countless tiny bobbins. Inactive DNA tends to be tightly coiled, whereas DNA containing active genes is more loosely wound and thus more accessible to the cell’s gene-reading machinery. A vast army of other proteins tweaks the histones to help regulate gene activity. Noh first became interested in the field of histone biology while pursuing her PhD at the Albert Einstein College of Medicine in New York, US. While studying the effects of stroke in the brains of rats, she found a protein that altered the histones in stroke-damaged neurons. She then began to investigate what was happening to the histones in cells that had permanently stopped dividing, making neurons a logical choice to study. Scientists already knew that actively dividing cells used regular, or ‘canonical’, histones whereas those that were paused before the next round of division used a different kind, known as ‘variant’ histones. But they knew very little about what was happening to the histones of cells that had stopped dividing permanently.
Variant histones seem to be associated with active regions of DNA, and so might have a specific role in regulating gene behaviour. This type of control would be especially important in long-lived neurons, which in addition to surviving a lifetime of wear and tear, also have to alter their gene activity in a highly dynamic way to respond to an ever-changing environment. During her postdoc at the Rockefeller University, Noh and her colleagues discovered that neurons that had ceased dividing did indeed incorporate variant histones into their DNA. But to really understand why, they had to find out when: did the variant histones creep in gradually, or were they added all at once?
To address this issue in humans, the team turned to radiocarbon. A technique known as accelerator mass spectrometry allowed them to tell the difference between variant histones containing regular carbon and radiocarbon. By studying post-mortem samples from people who lived through the atomic-test age, they found that the incorporation of variant histones seems to take place before puberty. “It’s not a gradual process,” says Noh. “A very robust replacement occurs during the early phase of human development, and the brain maintains the status quo over the course of the lifetime.”
These observations open up some very big questions. For example, what does this histone replacement during development really mean?
This suggests that histone replacement is a vital step in child brain development, coinciding with when the brain’s most dynamic learning processes are taking place, she explains. What’s more, recent genetic research has uncovered a raft of gene faults associated with abnormal brain development conditions such as autism and learning difficulties. Many of these genes are involved in histone biology. “These observations open up some very big questions. For example, what does this histone replacement during development really mean?”
Since joining EMBL in November 2014, Noh has been tackling these questions by growing neurons in the lab and performing a range of genetic experiments on them to work out what the histones are doing. This is easier said than done: one of the key challenges in the field of neuron biology is getting enough of the right type of cell to work on. So Noh’s team is taking immature cells from mouse embryos and coaxing them to form adult neurons in the lab dish. In addition, they are preparing to work on a kind of cell known as a human iPS cell, which they also intend to turn into neurons. These cells do not come from human embryos but from adult human cells that have been converted back into a more youthful state.
To alter the behaviour of the histones in lab-grown neurons, Noh’s team is gearing up to use a new technique called CRISPR. This allows scientists to ‘edit’ the content of genes in the cell by introducing changes into the histones of iPS-derived neurons. These changes, or mutations, will be based on ones known to play a role in human brain development conditions. The iPS studies will allow the team to explore the effects of these mutations on neuron behaviour.
Understanding more about neuronal histones could inform research into other conditions, including neurodegenerative diseases such as Alzheimer’s disease.
“Although the work is still in its earliest stages, understanding more about neuronal histones could inform research into other conditions, including neurodegenerative diseases such as Alzheimer’s disease,” says Noh.
Drugs directed at the cellular machinery that alters histones are now being used to treat certain cancers such as T-cell lymphoma, and studies of these drugs are providing new insights into how targeting histones could affect a cell. One emerging idea is that disease can result from DNA not being coiled tidily around its histones. “If you start to untangle all of this disorganised thread, the cell tries to find a way to rearrange it in ordered fashion,” says Noh. “Resetting a cell back to an ordered healthy state could represent a new therapeutic approach.”
Looking for past print editions of EMBLetc.? Browse our archive, going back 20 years.
EMBLetc. archive