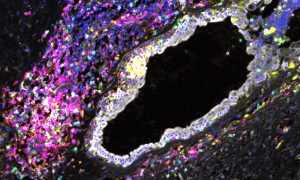
Read the latest Issue
Videos by scientists at EMBL Heidelberg showcase strength of the cell’s chromosome-separating machinery.
When it comes time for a cell to divide, ensuring that each daughter cell receives its appropriate share of genetic material poses a complex biological haulage problem. Large, unwieldy chromosomes have to be separated and pulled to opposite ends of the cell before they’re wrapped up into a new nucleus, and passed on to the next generation.
At the heart of this haulage operation is the spindle, a long mechanical device made of filaments that grabs hold of chromosomes and drags them through the viscous cytoplasm. Spindles must act precisely and yet have to be sufficiently strong. “In order to understand where that strength comes from, you have to look at the detailed architecture of the spindle,” says François Nédélec, group leader at EMBL Heidelberg, who led the study.
To get a detailed picture of the spindle in the yeast S. pombe, the team applied electron tomography to create computer models of the spindle’s architecture that shed light on how it does its job. Jonathan Ward, a postdoc in Nédélec’s lab, worked with Hélio Roque (now at the University of Oxford) from Claude Antony’s electron microscopy lab (now at IGBMC, France).
Looking at a living S. pombe cell that was frozen as it was about to divide, Nédélec and colleagues could follow the complete spindle (the purple and green lines) growing across the cell.
Using electron tomography, the team created models of spindles of different lengths: the three clips in this video show spindles ranging from around 3 to 9 micrometres – roughly the thickness of a strand of spider’s silk. Although it looks like each spindle is undulating like a wave, what you’re actually looking at is a rigid spindle rotating along its axis. “You can see that these spindles are not straight, but we’re not sure why,” says Nédélec. “Under a light microscope the spindles appear straighter, so they might be compressed by the high-pressure freezing used to prepare the cells for electron microscopy.”
These models reveal that as the spindles grow, they get thinner, with fewer microtubule strands: down from around 10 (five green, 5 pink) in the shortest to four or five in the longest. Nédélec’s team calculated that the amount of material in the spindle in fact remains nearly constant as it grows.
How strong does a spindle need to be to do its job? To explore this question, Nédélec’s team modelled spindles under stress. In this simulation, the spindle is subjected to increasing force, and by the time the spindle buckles, the forces surpass anything experienced in a natural cell. These models show that the longest spindles are just about strong enough to cope. “From an engineering perspective, it’s almost perfect,” says Nédélec.
Together, these studies and simulations provide a foundation for further studies of how the architecture of cellular machines in eukaryotic cells underpins the structural stability and mechanical power they need to do their jobs.
Looking for past print editions of EMBLetc.? Browse our archive, going back 20 years.
EMBLetc. archive