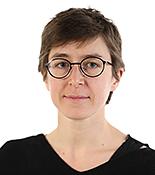
Anna Erzberger
Group Leader
ORCID: 0000-0002-2200-4665
EditTheory of cellular and multicellular organisation
Group Leader
ORCID: 0000-0002-2200-4665
EditLiving matter self-organises across temporal and spatial scales ranging from the molecular to the organismal. During embryonic development, for example, cells assemble into complex organs that reliably carry out specific functions. Understanding the principles governing self-organisation is one of the most important goals of both biology and the physics of complex systems.
Recent experimental advances have made it possible to observe the development of organs within living embryos, measure the forces exerted by cells, and track the exchange of molecular signals. This progress puts us in a position to leverage the theoretical machinery developed for simpler systems in traditional condensed matter towards studying the self-organisation of life. Moreover, the unique properties of biological systems necessitate theoretical innovations that could shift and change fundamental paradigms about the organisation of matter.
In our group, we combine approaches from soft matter and non-equilibrium physics and nonlinear dynamics to understand how large-scale phenomena emerge from the interactions and properties of their constituents in systems ranging from subcellular structures to embryos. We study how the spatial organisation of cells and tissues arises from feedback between molecular signalling processes and concurrent mechanical and morphological changes. In collaboration with experimental groups around the world, we apply our theories to explain and predict the observed behaviour of a diverse range of systems from cellular organelles to entire organs during morphogenesis.
Spontaneous generation of patterns and structures occurs in many living systems and is linked to biological form and function. Such processes often take place on domains which themselves evolve in time, and they can be guided by or coupled to geometrical features. Using different biophysical examples, we investigate how geometry affects spatial organization in cellular and multicellular systems. For example, relating the mechanics of embryo-uterus interactions during implantation to the physics of wetting, we uncovered how the right confinement geometry permits growing implantation-stage mouse embryos ex vivo (Bondarenko et al. 2023). Current topics of interest include i) boundary-induced alignment in anisotropic systems including polar tissues, ii) active surface dynamics and interactions with signalling processes (Dullweber et al. 2024, Erzberger et al. 2020, Dullweber and Erzberger 2023), and iii) mechanochemical pattern formation (Palmquist et al. 2022, Rombouts et al. 2023).
We propose a new formalism for describing Poissonian kinetics in discrete-state systems such as cellular Potts models, which takes into account both energetic costs and kinetic properties of a physical process. We rely on stochastic thermodynamics to separate thermal and athermal fluctuations, and to determine Poissonian transition rates between configurations of the system, which also introduce an unambiguous timescale into simulations (Belousov et al. 2023). These innovations permit i) investigating the role of active fluctuations without having to introduce a controversial notion of effective temperature, and ii) modeling the interplay of different timescales e.g. in composite materials with inhomogeneous transport properties. We applied our framework to dissect the effect of distinct active processes during developmental cell sorting – specifically cell growth and division versus active shape fluctuations – using live imaging data of isolated mouse inner cell mass (Moghe et al. 2023).
The nucleus is the largest organelle in most cells. Nuclear shape and position affects not only cellular mechanics and morphology, but also gene expression and signalling. We investigate the physical principles that govern nuclear shape dynamics in single cells, the feedback between large-scale tissue architecture and nuclear position in epithelial morphodynamics, as well as fluctuations and nuclear migration in pseudostratified neuroepithelia.