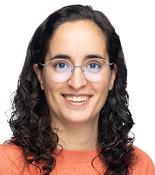
Alba Diz-Muñoz
Group Leader
ORCID: 0000-0001-6864-8901
EditMechanobiology at the cell surface
Group Leader
ORCID: 0000-0001-6864-8901
EditDespite being composed of a limited number of building blocks, biological materials exhibit a remarkable diversity of structures and purposes. For instance, keratin makes both tough structures like bird beaks and nails, and soft materials like sheep wool. To generate this multeity of shapes and functions nature often layers components. When subjected to external forces, the differential mechanics within a composite interface can drive morphogenesis at a broad range of length scales.
In animal cells, the cell surface is a composite interface, comprising the plasma membrane, the underlying cortical cytoskeleton, and the ‘glue’ that binds them together — the membrane-to-cortex attachment (MCA). The resulting cell mechanics is not just the sum of these various components, as these layers are mechanically coupled in non-intuitive ways. Furthermore, cells do not exist in isolation within organisms; feedback mechanisms also exist with neighbouring cells and the extracellular matrix.
We are only beginning to understand the logic of how different cell surface mechanics crosstalk to control form and function. Current approaches to measure and manipulate forces have important limitations, highlighting the need for and new tools and techniques. Our lab works towards deciphering the molecular and mechanical principles underlying complex behaviours such as cell migration and differentiation. We study cellular force transduction in an unprecedented and innovative manner by combining multidisciplinary approaches and developing creative experimental tools to measure and control cellular forces at the cell periphery. Moreover, our multiscale approaches feed theoretical frameworks to decipher how a composite interface gives rise to complex behaviours.
The lack of specific tools that modify particular physical properties and bridge molecular-to-cellular scale biophysics prevent us from understanding how cells use their mechanics to govern function. Combining an engineered mechanical membrane-to-cortex linker with atomic force spectroscopy measurements of membrane mechanics allowed us to show that tethering the plasma membrane to the cortical cytoskeleton controls the exit from naïve pluripotency in embryonic stem cells (Bergert et al., 2021). Moreover, in a recent preprint, we for the first-time link cellular mechanics to the molecular structure of the cytoskeleton, as revealed by in situ cryo-electron tomography. This allowed us to identify a novel and unexpected mechanism for the control of cell mechanics: the regulation of the nanoscale distance between the plasma membrane and the actomyosin cortex (Lembo, Strauss, et al., 2023).
The mechanical feedbacks at the cell periphery give rise to an incredibly diverse topography at the surface of animal cells, which in turn also encodes key information for processes like cell motility. In a recent preprint aimed at understanding how plasma membrane curvature controls motility, we show that a cell’s persistence and directionality strongly depend on the activity of Snx33, a BAR domain protein (Sitarska, et al., 2021).
The environment that surrounds a cell is key for development, physiology, and pathology. A cell, its neighbours and the extracellular matrix can also be seen as a composite interface. On that front, we have taken advantage of the imaging possibilities in the zebrafish embryo to reveal how gene expression patterns result in the generation of different cell types (Sanchez-Iranzo et al., 2022), and in a recent preprint probed the lipid metabolic landscape of developing neurons (Gopalan et al., 2022). Also, through interdisciplinary collaborations, we have shown how fibroblast-induced matrix stiffening influences prognosis and therapy in colorectal cancer (Shen et al., 2020a and 2020b). This surprising discovery highlighted that (i) currently used drugs have unknown mechanical effects worth exploiting therapeutically and (ii) modulating the mechanical microenvironment of tumour cells can significantly improve therapeutic regimens. Lastly, together with the Prevedel lab, we have significantly advanced Brillouin microscopy to observe extracellular matrix mechanics in tissues in vivo (Bevilacqua, et al., 2019, Sanchez-Iranzo, et al., 2020, Bevilacqua et al., 2022).
Together, our work provides new insights into how cells couple physical forces and material properties to drive essential processes, underscoring the importance of exploring the role of composite interfaces in development, physiology, and pathology. In our future work, we will build on these exciting results and expand our toolkit to mechanically program the cell surface.
We will continue to use in situ cryo-electron tomography and atomic force microscopy to determine the structure-function relationship at the cellular periphery in space and time. Moreover, we will leverage state-of-the-art light and electron microscopy, combined with novel optogenetic tools, to further explore how the topography of the plasma membrane is used for cell migration and membrane trafficking. Furthermore, we will adopt an unbiased multi-omics approach to decipher the origins of tissue elasticity and its effects on immune cell migration. Lastly, our work will continue to provide the quantitative data needed to build theoretical models and ultimately decipher the physical principles underlying morphogenesis and differentiation.