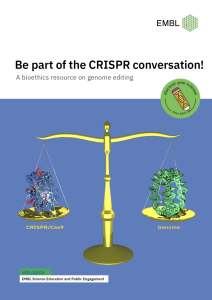
Formerly known as European Learning Laboratory for the Life Sciences
Our inspiring educational experiences share the scientific discoveries of EMBL with young learners aged 10-19 years and teachers in Europe and beyond. We belong to EMBL’s Science Education and Public Engagement office.
Dr Merle Hantsche-Grininger sheds light on what it’s like working at the forefront of cell biology research
Leading up to our latest virtual LearningLAB featuring Dr Jan Ellenberg as a keynote speaker, Hariharan Arevalagam sat down with Dr Merle Hantsche-Grininger, a postdoc at the Ellenberg Group at EMBL Heidelberg, to talk about her research, what the life of a scientist is really like, advice for students, and much more.
Merle is a biochemist, and her focus in the Ellenberg Group is chromatin architecture; making chromatin visible by developing a method that allows the labelling of DNA and the proteins that define its compactisation. Before getting into the details of her research, she tells Hariharan about her experience as a high school student.
Let’s travel back in time, and talk about your high school days. What drove you to pursue biology to begin with or science in general? And what did that mean to you as a high school student?
My interest in biology and biochemistry, especially biochemistry, developed in the last few years of high school. In school, at least for me, I often had this experience that how much I liked the subject was very dependent on the teacher, and I had very nice biology and chemistry teacher, who actually was a biochemist, which I learned later on.
This is why I decided to study biochemistry. Not because I wanted to become a scientist but because the subject was very interesting to me; I was very fascinated to learn how a cell works and how so many cells work together to form an organism.
At school, I didn’t have a clear picture of what I wanted to be in ten years. I wanted to do something that gives me the freedom to decide what I want to do later on – something I, of course, enjoy doing but still isn’t limiting my decision to go to a completely new field afterwards. With biochemistry, you can do many things after you graduate: go into academic science or industry, you can do consulting, you just learn this kind of logical thinking as a scientist. And this way of approaching things gives you a basis to do many different things later on. It doesn’t limit your career path, but it rather opens up more possibilities. That was important for me. I didn’t want to determine my future when I was 19.
What were your next steps after high school?
I finished high school in Bonn [in Germany], and after my Abitur [the German equivalent of A-Level], I moved to Tübingen to study biochemistry. During my studies, I did a lot of internships in and outside of Germany. I went to Madrid for a few months and to Munich to do several internships. I then decided to do my diploma thesis in Frankfurt am Main. Afterwards, I decided to do my PhD in a structural biology lab in Munich. During my PhD, I was expressing and purifying proteins involved in transcription- one of the processes involved in gene expression. In the beginning, I used X-ray crystallography to see the atomic structures of these protein complexes interacting with DNA. Later, with cryo-electron microscopy, we could see bigger structures and larger complexes that could show us how the initiation of transcription is regulated.
How did you end up working in EMBL?
In structural biology, you want to get a high-resolution structure of a certain protein or protein complex. This can only be achieved if you look at this protein in an isolated system – you purify the specific protein to the extent that it is so pure that you are able to look at its individual atomic components. For me, the next step was to look at the process in its cellular context, where components work in harmony and aren’t stripped away from their partners and their surrounding. To do that, I think that one of the best methods currently available to understand gene expression on a cellular level is super-resolution microscopy. It is an advanced form of fluorescence microscopy and allows you to look into cells with a resolution of tens of nanometers. Super-resolution microscopy allows you to visualise big structural arrangements or biochemical processes and see where and when they occur inside the cell.
This is why I joined Jan Ellenberg’s group at EMBL in late 2017 for my postdoc. Currently, I’m trying to visualise and understand a critical aspect of gene expression using super-resolution microscopy.
Can you tell me more about what your specific research is about? How does your research contribute to the bigger picture, and what is the bigger picture anyway?
I’ll start a bit broader here: DNA does not exist naked but exists in an intricate complex with proteins, forming the so-called chromatin. These proteins organise our DNA on many levels. But chromatin organisation is still a mystery.
To start with, in each cell, there are two metres of DNA. If we unwind our chromosomes and then line up the DNA double helices next to each other, it would be two metres long. The diameter of a cell nucleus, depending on the type of cell, is just a few micrometres and this long stretch of DNA fits into it. To give an analogy, it would be like trying to fit a fishing line that’s 200 km long into a soccer ball. Even more fascinating is that all the cells in our body are different; we have skin cells, liver cells, eye cells, and hair cells, but they all contain the same DNA. The spatial organisation of the DNA determines which genes are expressed in each cell, which in turn determines the cell’s fate, or what we call phenotype.
Think of it like this: the nucleus is considered the “library” of the cell. The books are the information carried in DNA. The shelves are the proteins. Depending on how the library is arranged, you might only have access to certain shelves and books, not others. Differently arranged libraries can be found in the different types of cells. Understanding chromatin organisation gives us an understanding of how a cell organises all the DNA information that is the same in all cells by how it arranges these metaphorical bookshelves.
So, what I am currently doing is to purely gain knowledge about something that we don’t understand. If we understand the principles of chromatin folding, this has, of course, implications for medical and pharmaceutical applications. Personalised medicine, for example, is highly dependent on understanding your DNA and what kind of potential diseases you might get.
The topic of chromatin organisation nicely illustrates that scientific advancement and insight always depend on the methods and techniques that we have available. A few years ago, we had X-ray crystallography that let us look at details on a very small scale of nuclear organisation, and we had light microscopy that let us look at things on a bigger scale. So, we could see chromosomes, but everything between chromosomes and nucleosomes was invisible. There was no microscopy method that looked at the “in-between” scales of chromatin organisation. Now, with super-resolution microscopy, we have the techniques and the possibility to look at these finer scales inside the cell. And this is the very first time we can do this! This is what makes it interesting because no one has seen what chromatin looks like at this resolution. So, what we know always reflects the current state of technology.
How does it feel to be at the frontier of knowledge?
It’s great! It’s so exciting! Because after having finally figured out all the technical details, I now have a super-resolution image of this chromatin organisation, and I know I’m the first person in the world looking at this. It’s just really cool!
Where do you see the future of your field? Based on our conversation, I don’t think we’re ever going to be done with this scientific endeavour.
I think we are never done. That’s the beauty of it, right? With every open question that we answer, ten more arise. The genome is the essence of our body, and understanding nuclear organisation will help us understand what life is. I think we’re all driven by the essential questions, the big questions: What is life? What defines life? How do these millions of cells communicate, and how do we develop a personality from all these biochemical processes? These are fundamental questions, and I am working on unravelling a very fine detail relevant to one of these questions. But if we understand many small details, we can put them together, and that’s the fun part! You have many people, thousands of research groups worldwide, working on many small details. It is so rewarding when you can put the small details into a bigger picture and start to understand how everything comes together.
Could you tell me about a challenge that you had to overcome? Or a failure that you faced and how you bounced back from it? Not only as a postdoc but in your career in science in general.
There are failures and challenges on many levels. Being at the frontier of doing something new means that nothing works as expected.
Even on a daily basis, you already have so many failures. For example, it takes me a week and a half to prepare my cells for imaging at the microscope. And then you’re finally at the microscope, and the microscope is broken. This happens. Something might break, and you just have to start over.
But then, of course, there are bigger failures. For example, I first wanted to study at the ETH Zurich, and I applied, but I didn’t get a spot. But I did get a spot in Tübingen, and I met so many amazing people there; I met friends for life. And then, of course, you have failures like when you’re applying for fellowships, and you’re not selected. But there will be a time you get lucky and are successful. So yes, I think these are the failures and challenges that everyone encounters doing science and the only way to overcome them is to stand up again and just continue.
How do you keep yourself motivated throughout all this? Especially with the daily challenges.
It’s tough. But when something does work, it’s so rewarding. When you get your image or your protein structure or your publication! Things like these motivate you to go through all of this.
Media representation is not very accurate when it comes to scientists. To show how a scientist actually is, I think it would be helpful to talk about your daily routine and what you do outside of work.
I have a two-year-old son, so part of my life outside of science is my family. My daily routine is to bring my son to the nursery and go to work. With a family, you’re much more restricted with the time you have for work, but I feel that I am much more effective and efficient with my work. I also enjoy sports of all kinds – cycling, running, downhill and cross-country skiing in winter. I like to cook, as well. Of course, meeting friends is not so easy with kids anymore, but these are things that all parents face, whether in science or not.
The myth of the lonely scientist is not necessarily true. Actual scientists don’t work alone, isolated from everyone else. As Hariharan stepped into Merle’s lab to meet her, he saw many other people at desks and benches working away at their own tasks while also jovially talking to each other.
When I visited your lab, I saw multiple people working on different things. They have different roles, right? Could you explain how that works in a lab?
You have scientists at different stages [of their career] in one lab. When you’re a student still in university, in order to graduate, you have to do your bachelor’s thesis and then your master’s thesis. To do those, you go into scientific labs and work with a supervisor on your project. So you have students in the lab, like master students or interns. For example, when I did my studies, I had to do several internships where I could choose which labs I wanted to go to, and I worked there and learned laboratory and research techniques.
Then you have the PhD students. When you want to do your PhD, you choose a lab, and you get your own project. A PhD takes three to four years to finish. When you’re done with your PhD, and you have defended your PhD thesis, you can stay in the same lab or choose a different one to continue working as a postdoc.
And you also have technicians. Sometimes they help prepare general things needed in the laboratory, but we also have technicians who themselves have a PhD or any other science education, and they would support PhD or postdoc projects.
In our group, you wouldn’t be able to tell from the outside who’s a postdoc and PhD.; We all work very closely together and discuss our research with each other a lot.
Finally, there is the Principal Investigator (PI), who is in charge of the lab and who mentors PhDs and postdocs.
It sounds like being a scientist is a social job.
Exactly. And if you were to ask me what kind of qualities you would have to bring to work in science, there are no specific qualities because all people working in science have different characteristics. They can all be successful.
The more different personalities there are within a team, the more creative the team is. If all my colleagues think the same way as I do, problem-solving gets very difficult. It’s very good to have people with different backgrounds, with different education, but also from different countries. It’s good to exchange with people who think differently because they can show you aspects of your work you haven’t thought of. We usually have a certain way of approaching problems, and it’s always difficult to step back and look at things from a bigger perspective.
So there’s nothing specific you have to bring with you to science. But I think what would make your work and life much easier is if you like to collaborate closely with others and like to talk to people. I think this is very important in our daily life as scientists.
Okay, and should we be trying to get more kids interested in science?
Definitely!
Why?
It’s related to what I said earlier about teams. We need more kids interested in science to be more creative. We need more people from different backgrounds, and we need more women. During university studies, the ratio between female and male students is more or less 50/50, or maybe even slightly more female students. At the PhD level, it’s still very similar, but at least in academic research, the higher you go up the career ladder, the fewer female scientists you’ll find.
The leaky pipeline, right? What do you think we should be doing to address that?
For me, having examples and role models helps. I think it’s very important for students, especially female students, to see that being successful in a certain academic career, e.g. as PI, and having a family IS possible and that it is not the exception. If more people from diverse backgrounds are in science and have group leader positions, the students can identify with them and think, “This person is just like me, they are successful and leading their own group. This is what I want to be when I’m older.” I think that will help.
Is there any advice you would give a high school student who is thinking about going in this direction but isn’t really sure?
I would say: just go for it. Get started. You can always decide to go in a different direction or take another path, and you wouldn’t lose anything. It will just give you more opportunities and more possibilities. Nothing is fixed and final. Go step by step. You can always change your path, so just go for it!
Chromatin | A DNA-protein complex that organises eukaryotic DNA into a highly compact form. |
Chromosome | A packaged and organised structure which contains most of the DNA of a living organism. In plants and animals, it resides in the nucleus of cells. Humans have 22 pairs of autosomes and one pair of sex chromosomes (XX in women and XY in men). |
Cryo-electron microscopy1 | Technique for observing flash frozen (-196ºC) specimens using an electron microscope. |
Electron microscope | A microscope that can be used to observe an object with high resolution by applying an electron beam instead of a beam of light, which is used in conventional light microscopes. |
Gene expression | A process in which genetic information is used to synthesise a functional gene product (a protein or a non-coding RNA). |
Fluorescence microscopy | An imaging technique that uses a special microscope to observe objects that give off fluorescent light. This light can be produced by the object (autofluorescence) or come from a dye that can be used on samples which don’t give off this light naturally. The microscope uses light of a specific wavelength to excite the objects or the dye, which lights up and allows us to view the sample. |
Nucleosome | For the almost 2 m long DNA present in every cell to fit inside the nucleus, it must be packaged in a specific way. A nucleosome is the basic subunit of this packaging, forming the structure of chromatin. It comprises 145–147 bp of DNA wrapped around a core made of proteins called histones. |
PhD/Doctoral studies | Following their undergraduate or postgraduate studies, students can continue their education at the next academic level to conduct original research projects. Upon defending their PhD thesis before the University committee, students are awarded a “PhD” or “Doctor of Philosophy” title. |
Postdoc | A postdoctoral researcher, commonly referred to as a “postdoc”, is a researcher who already has a PhD or an equivalent degree and carries out further research to continue their academic career. |
Protein expression | A series of cellular processes that lead to the production of a protein product from the information contained in the DNA. In the lab, scientists can produce proteins of interest in large quantities (protein overexpression), such as insulin. The proteins are then subjected to several purification steps to obtain highly pure proteins that can be used in follow-up experiments and later applied in medical treatments and diagnostics, for example. |
Super-resolution microscopy2,3 | Field that encompasses fluorescence imaging techniques with the capability to visualise objects with extraordinary detail. |
Transcription | It’s the first step in gene expression, where the enzyme RNA polymerase uses DNA as a template to synthesise a complementary RNA copy. |
Translation4 | The second step in gene expression, during which the ribosome machinery translates the information in the messenger RNA following the genetic code. This code relates the DNA sequence to the amino acid sequence in proteins. |
X-ray crystallography | A method of determining the arrangement of atoms within a crystal, in which a beam of X-rays strikes a crystal and causes the beam to spread (diffract) in many specific directions. From the angles and intensities of these diffracted beams, a crystallographer can produce a three-dimensional picture of the density of electrons within the crystal. From this electron density, the positions of the atoms in the crystal can be determined, as well as their chemical bonds. X-ray crystallography is used to determine the atomic structure of many materials, such as salts, minerals and metals as well as organic and biological molecules such as proteins and drugs. |
Clancy, S. & Brown, W. (2008) Translation: DNA to mRNA to Protein. Nature Education 1(1):101 https://www.nature.com/scitable/topicpage/translation-dna-to-mrna-to-protein-393/
The research carried out by the Ellenberg Group forms the core research topic of our upcoming virtual Learning LAB, “The cellular basis of life”. For more information on how you can participate, head on over to the course page here.
Topic area: Cell biology
Type of resource: Interview
Age group: 16-19
Author: Hariharan Arevalagam
Share: