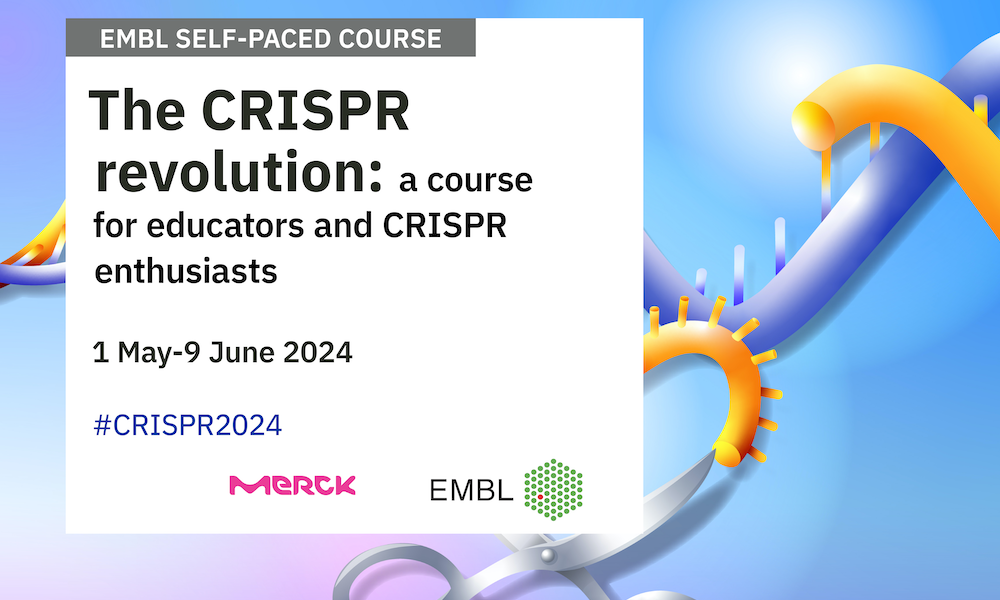
Formerly known as European Learning Laboratory for the Life Sciences
Our inspiring educational experiences share the scientific discoveries of EMBL with young learners aged 10-19 years and teachers in Europe and beyond. We belong to EMBL’s Science Education and Public Engagement office.
ELLS Guest Blog by Kanchan Anand
As a fresh outbreak of Ebola takes its toll in West Africa, I ask myself why we know a lot about viruses and yet do not have a vaccine or a drug against a lot of them. A little over two hundred years ago, in 1796, Edward Jenner developed the first antiviral vaccine to treat smallpox virus and since then scientists have developed vaccines for various viral diseases such as measles, mumps, rubella, polio, hepatitis, influenza and rotavirus. However, we still have no vaccines against the common cold and many other, often deadly, viral infections such as Lassa, Marburg, SARS and H1N1. This seems perplexing at first, but let’s take a closer look into why handling a virus is not just challenging but actually quite tricky. There are a number of reasons, the first of which is that they are usually very small in size (ca. 20 – 250 nm). Their small size is illustrated by the fact that some of them have the ability to infect even the tiniest bacteria – in the case of bacteriophages. Secondly, viruses are not susceptible to antibiotics. And thirdly, their life cycle involves invading the host cell by fusing spontaneously with the host cellular membranes. This allows them to transfer their own genetic material into the cell, to multiply and to make it very difficult for the host cell to function in a normal manner. Finally, continuous genetic shifts and drifts in viral genomes modify viruses in a pace which vaccine development often cannot keep up with. This is one of the most prominent reasons why it is so difficult to control recurring influenza outbreaks.
In order to produce a vaccine against influenza (or any other virus), scientists need information of two worlds: about the virus and about the process of drug development. Viruses are a diverse group of infectious agents and might be of different kinds, shapes and sizes For example, there are double and single-stranded DNA and RNA viruses (e.g. influenza virus) as well as reverse transcribing viruses (e.g. HIV), which can adapt very effectively to their host cells. Viral structures can be helical, isocahedral, prolate, enveloped by membranes and even more complex. Viruses not only infect humans but also animals, plants, archaea and bacteria. In humans and animals, viral infection can be found on any part of the body, although it most commonly occurs in the intestine and the upper respiratory system, i.e. lungs and airways. Statistically speaking, the most common symptoms of viral infections in humans are fever, headache, fatigue, diarrhea and a running nose.
How can structural biology help with anti-viral drug development?
In-depth knowledge of the respective virus and its viral coat protein structure is a prerequisite for developing many anti-viral drugs which have the long-term ability to be effective against different classes or subtypes of a specific virus. Based on high resolution structural data and detailed knowledge of functional mechanisms of a virus, scientists can devise possible strategies to inhibit one of the following essential steps of the viral life cycle: viral entry into host cells, the genome replication process, viral assembly or the release of viral particles, which spreads the infection. For example, based on the crystal structure analysis, data scientist could locate the neuraminidase active-site and develop drugs against few strains of influenza virus: Oseltamivir or Tamiflu® (Gilead Sciences & Genentech) to treat avian flu virus infection and Zanamivir or Relenza® (Biota & Glaxo SmithKline) to treat the Spanish flu virus.
Finding an answer to the question if we will ever win the race to combat all types of viruses is not straightforward. Drug development strategies work for some viruses but not for all. Many viruses (e.g. influenza virus) keep changing and every decade or so, come up with a dangerous new strain presenting a potential risk for public health. For instance, although there is plenty of scientific literature on the influenza virus and as short term vaccines are available, we still do not have a drug to treat infected patients. The initial symptoms of influenza virus infection are similar to the common cold and stomach flu infection. This makes early detection by symptoms difficult and once the infection is detected it might be too late for antiviral drugs to effectively contain the infection. The transmission of influenza virus occurs in three different ways: (1) direct, (sneezing or coughing by infected people can transmit the virus particles into the eyes, nose or mouth of non-infected people), (2) aerial course (inhaling the aerosols of an infected person) and (3) by touching contaminated surfaces, where the virus can stay active for hours. Symptoms appear mostly after the third day of infection and are characterized by high fever. Like the common cold, influenza is also seasonal and affects millions of people around the globe, out of which about half a million die (source: WHO) as result of the infection. An obvious question that arises is the following: why do we not take the vaccination before it is too late? The reason for this is the fact that the virus mutates at a very fast rate which means scientists have to predict and agree which of the mutations could lead to an epidemic. Based on this information the influenza consortium at the World Health Organization decides on the yearly production of influenza vaccine(s). Developed nations have the resources to offer a flu-shot against the “currently circulating form of influenza virus” to a majority of the population but this short-lived vaccination becomes ineffective the following year as the virus evolves rapidly. Like all RNA viruses, new influenza strains quickly replace their older ones, making subsequent treatment difficult. Genetic recombination, shuffling of genes or mutations among different species can result in deadly viral infection. For example, swine-flu (influenza A/H1N1) which emerged in Mexico and several countries in 2009, evolved from combined genes of influenza viruses infecting humans, pigs and birds.
The intriguing mechanism of viral replication excites many researchers to solve the biological puzzle of stopping the infection(s). Therefore, this is a fascinating field of science with huge research potential toward therapeutics especially if one works in developing a way to predict the next possible mutation and be ready in advance to obtain a vaccination. With the latest technologies, experimental data, previous experiences and highly motivated researchers we hope that scientists will soon outsmart viruses and eventually virus wars could be overcome.
Information about the author: The author is a protein x-ray crystallographer. Although her main interest is in viral proteins, she is working on structural aspects of several different proteins that are therapeutic targets.